Many policymakers have traditionally seen climate models as irrelevant, but Gavin Schmidt argues that recent advances are making such models an essential tool in informing policy choices
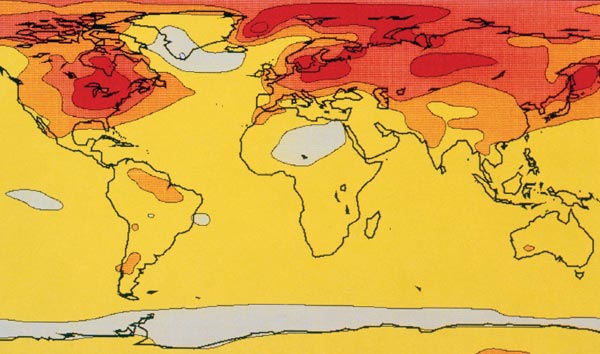
A quick tour of the Internet reveals some very strong feelings on the subject of climate models. Unsurprisingly, on climate contrarian sites, such models are described in all sorts of unflattering terms and dismissed out of hand as fundamentally useless. However, in more rational forums, and sometimes even among scientists themselves, one occasionally comes across a basic ignorance of whether climate models are any good, and, even more importantly, what they are good for. By the time one gets to policymakers, climate models are seen at best as black boxes, and at worst as simply irrelevant to their detailed concerns. However, climate models – appropriately used – might have a vitally important part to play in breaking through some of the log jams now hampering policymakers.
The complexity of climate
Models of any stripe are simply quantitative or numerical expressions of the theories we have for how the real world works. Climate models encapsulate what we know about how the Sun’s rays travel through the atmosphere and how heat from the surface of the Earth gets absorbed by clouds, water vapour and, of course, carbon dioxide. They contain sophisticated (though imperfect) representations of cloud formation and rainfall, floating sea ice and ocean turbulence, rivers and lakes, and soil and vegetation. Each representation is based on direct observations of the processes in question and is tested against many different constraints. The models (and there are many) have numerous common behaviours – they all cool following a big volcanic eruption, like that at Mount Pinatubo in 1991; they all warm as levels of greenhouse gases are increased; they show the same relationships connecting water vapour and temperature that we see in observations; and they can quantify how the giant lakes left over from the Ice Age may have caused a rapid cooling across the North Atlantic as they drained and changed ocean circulation patterns.
This gives us a hint: models are useful for tying together causes and effects in complex systems where answers are often only obvious in hindsight. We can apply them for climate changes in the past – global changes in temperature or rainfall patterns inferred from the paleoclimate data for instance – and help attribute events to causes. Indeed, the attribution of any particular climate trend or set of events is inherently a model-based exercise. Without a way of telling the difference that any particular cause might have, how can we recognize its fingerprint in the real world? This is the basis of the conclusion of the Intergovernmental Panel on Climate Change (IPCC) that human activities are behind the rise in global temperature in recent decades.
The other use is in helping chart the course of the future. People are quick to dismiss model projections of climate as being inferior to observations, but as Tom Knutson and Robert Tuleya, from the Geophysical Fluid Dynamics Laboratory at Princeton University and Old Dominion University, Virginia, pointedly noted in 2005, “If we had observations of the future, we obviously would trust them more than models, but unfortunately observations of the future are not available at this time.”
However, prediction is hard, particularly of the future (to paraphrase Niels Bohr). For the climate, there are two kinds of possible predictability. The first is based on extrapolating seasonal and interannual changes based on precise knowledge of today’s state of the atmosphere and ocean combined with an understanding of how the various modes of variability in the ocean might develop. Whether these efforts can provide useful information on regional climate on year-to-year and longer timescales is currently being explored. The more usual source of predictability, however, is considering the long-term changes related to increases in greenhouse gases, a volcanic eruption or other changes in the composition of the atmosphere. The first relies on a thorough understanding of patterns like El Niño or the North Atlantic ocean circulation, while the second tries to average over that variability to predict changes in the mean state.
For this second kind of prediction, you always need a scenario for what might happen to the drivers of climate change. Will carbon dioxide concentrations continue to increase? Will air pollution continue to decrease in the developed world but increase in the developing world? How fast will tropical deforestation progress? These scenarios are highly dependent on economics or political decisions and so qualify easily for the “hard prediction” category. Nonetheless, economists do their best to make a range of reasonable estimates for plausible futures and calculate the resulting changes in emissions.
But climate is complex. There are multiple causes, giving rise to multiple effects such that the interactions among the various components – like low-level ozone, aerosols (airborne particles) and clouds – can get hideously complicated. Ozone near the ground is created from the soup of emissions from car exhausts, factories and fires, and it is a public-health problem as well as a greenhouse gas. Aerosols too can come from multiple sources: sulphur-dioxide emissions from coal-burning power plants produce sulphate aerosols in the air; black carbon (soot) and organic-carbon aerosols come from incomplete combustion of biomass and even from the complex organic molecules emitted by plants. They all interact directly with the Sun’s radiation to either block it (for sulphates) or increase absorption (black carbon). They also have indirect effects by changing how easy it is for clouds to form, or by changing how reflective snow is (black carbon effectively makes the snow dirtier).
Solving the puzzle
Science, however, has made tremendous progress by trying to break things down into their component parts. Thus, we have traditionally studied the impact of carbon dioxide separately from the impact of sulphate aerosols and separately from the impacts of the emissions that cause ozone (the “precursors”). Frequently, these studies are carried out by separate scientists, in separate institutions under separate grants and with separate goals. While this has led to a great deal of insight, it has also tended to divorce the science from policy.
Let me give some examples. In the last IPCC report there is an iconic figure that shows the magnitude of the effects on climate for the 20th century. Carbon dioxide is the largest warming factor, followed by methane, nitrous oxide, low-level ozone and black carbon. On the cooling side, there are sulphate and nitrate aerosols and land-use changes. There is nothing wrong with this picture, but how helpful is it in deciding what to do about the power generation in China that produces carbon dioxide but also sulphates, or for assessments of mileage standards that will affect ozone precursors, black carbon from diesel exhaust as well as gasoline use? In each case, we have mixed results for the climate and potential impacts on other public-policy issues as well (air quality for instance). Because of scientists’ focus on single-factor experiments (change carbon dioxide, or change black carbon, or change sulphates), we have not historically provided enough information for policymakers to properly weigh up these different effects. Neither have we clearly identified the key sectors around the world that might provide win-win-win scenarios for people worried about climate, air quality and ecosystems.
However, scientific and computational advances in climate modelling and validation over the last few years now mean that we can do a much better job. Models now include many more of the interactions that matter: atmospheric chemistry that can predict ozone concentrations as a function of the methane or carbon-monoxide precursors; or aerosol physics for multiple kinds of particles – those directly emitted, like soot and mineral dust, and those created in the atmosphere from other emissions. More importantly, the models now include myriad interactions: the chemistry that takes place on the surface of dust aerosols that in turn affects sulphates; the impact of increasing methane on atmospheric oxidation, which affects aerosol concentrations; or the affects that aerosols have on clouds or snow albedo.
We can therefore now start to directly answer the questions that policymakers are raising – and some of the results may be surprising.
In Europe, for instance, the use of coal for power generation produces very little sulphate aerosol or black carbon because of existing air-quality controls. Thus, the only options for reducing the climate impact of coal relate to specific reductions in coal burning or investment in carbon capture and sequestration.
However, in India and China a lot of coal and biomass is burned in domestic settings where inefficient low-temperature combustion and a lack of pollution controls mean that the mix of emissions is much more complicated – carbon dioxide, of course, but also large amounts of carbon monoxide, black carbon and sulphates. Together, these emissions contribute strongly to the “Atmospheric Brown Cloud” phenomenon and to the appalling air quality in the region. This implies that efforts to improve rural electrification for instance – even if the power is generated in a modern coal power plant – could still reduce net climate warming because of the impacts on reducing ozone, methane and black carbon. These kinds of strategies are already being pushed by the Indian government because of the more direct impact on indoor and regional air quality and to reduce the deforestation associated with biomass collection, but a recognition of the net climate impact may help bridge the current gaps in the international negotiations on a climate treaty.
Other surprises include the recognition that reducing methane emissions from whatever source has important indirect impacts on a range of other drivers and is a more effective strategy for short-term reductions in global warming than had been previously recognized. As we move forward, we should be able to assess the net climate impact of any particular policy given the changes in emissions that will result.
Like a full life-cycle analysis for judging the impact on net emissions of a switch in energy-generation technologies, a full Earth-system analysis should become the new standard in judging climate-policy proposals.
All climate models are wrong, but some of them are useful, and by working more closely to answer the questions that are actually being posed by policymakers, we can make them more useful still.