
Quantum computers are a study of extremes. On the one hand, they promise to be far more powerful than classical machines in solving certain problems. On the other, their quantum nature is remarkably fragile and sensitive to environmental noise. To perform scalable, useful quantum computations, therefore, scientists need to correct these errors in an efficient way. One important step towards this goal is to perform quantum computations in a way that stops correctable errors from spreading (and thus becoming uncorrectable). Now, for the first time, researchers in Austria and Germany have experimentally demonstrated a universal set of these so-called fault-tolerant quantum operations, laying the groundwork for large-scale, error-corrected quantum computation.
Error correction
The key to any sort of error correction is adding redundancy to the information that needs to be protected. A classical computer, for example, might make several copies of each bit so that if any one bit incorrectly changes value, looking at all the bits at the end of the computation gives you a very good idea of what its value should have been. In the quantum case, copying and checking information is more complicated, but the idea of adding redundancy is the same: data contained in a single physical qubit is spread among many qubits using entanglement, in a way that lets the machine both detect errors should they happen and perform corrections without compromising the state of the computation. These many physical qubits are referred to as a logical qubit and the entangling transformation is known as encoding.
If keeping the state of the logical qubit unchanged forever were all anyone cared about, error correction would relatively straightforward. The real difficulty arises when the state of this large composite system needs to be manipulated, since each step of the quantum algorithm now has to be performed on the many physical qubits that encode one logical qubit. This adds errors and creates additional problems, as not all quantum operations are straightforward to translate into this large logical form. Notoriously, an important quantum operation known as a T-gate requires complex and resource-heavy methods to perform in a fault-tolerant way – to say nothing of multiple-qubit interactions.
Complete set of building blocks
In the latest work, which is described in Nature, researchers from the University of Innsbruck and RWTH Aachen University demonstrated a full set of fault-tolerant quantum operations on an ion-trap quantum computer. As well as implementing a T-gate, the set includes preparing qubits in configurations known as logical Pauli eigenstates and creating an entangling CNOT gate between two logical qubits. These ingredients allow a single qubit to be transformed to every possible state and to interact with other qubits, making them the basic building blocks of any arbitrary quantum computation in the fault-tolerant setting.
The qubits in the experiment are encoded in electronic states of 16 calcium ions suspended using magnetic fields (a macroscopic Paul trap) and controlled with individual laser beams. The advantages of using trapped ions instead of the superconducting qubits favoured by many quantum computing firms include lower error rates and better connectivity between qubits, though there are challenges with scaling the technology. The researchers use seven physical qubits to make each logical qubit via an encoding known as the colour code, and their system also incorporates additional physical “flag” qubits to signal the presence of dangerous errors in the system. This technique drastically reduces the resources required for error correction. Importantly, the experiment demonstrates that the fault-tolerant scheme performs better than a non-fault-tolerant implementation, despite the additional complexity in the underlying algorithm.
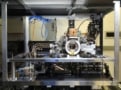
Physicists achieve fault-tolerant control of an error-corrected qubit
The results mark a big step forward for fault-tolerant quantum computing, although many more steps remain before truly large-scale experiments can be carried out. “Encoding more logical qubits – either by using more small error correction code or by using codes that host multiple logical qubits in one entangled multi-qubit state – is one of the next goals,” says Lukas Postler, a PhD student at the University of Innsbruck and one of the authors of the paper. “A short-term goal certainly is to develop a more refined error model for a better understanding of the error processes and their effect on the logical qubit performance,” he adds. As well as modelling errors and implementing more logical qubits in the short term, in the long term the researchers hope to accomplish repeated rounds of error correction on the system, which are indispensable for large-scale quantum computation.