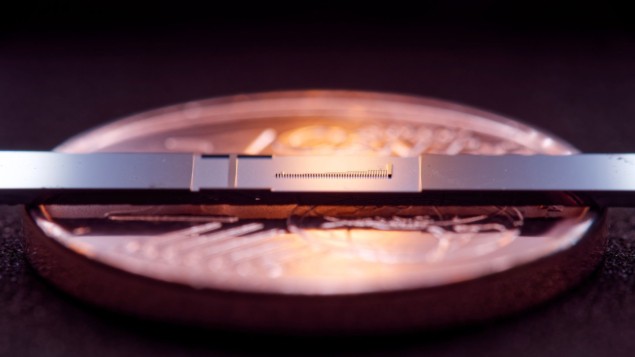
Laser-driven particle accelerators on silicon chips have been created by two independent research groups. With further improvements, such dielectric laser accelerators could be used in medicine and industry – and could even find application in high-energy particle physics experiments.
Accelerating electrons to high energies is normally done over long distances at large and expensive facilities. The electron accelerator at the heart of the European X-ray Free Electron Laser in Germany, for example, is 3.4 km long and the Stanford Linear Accelerator (SLAC) in California was 3.2 km long.
As a result, the use of electron accelerators for practical applications in medicine and industry is severely restricted. Size and cost are also factors in accelerator-based particle physics, where facilities are getting bigger and more expensive as they reach for higher collision energies.
Surfers on a wave
In a conventional accelerators, microwave oscillations of electric fields in metallic cavities accelerate electrons like surfers on a travelling wave. The maximum acceleration gradient is typically a few dozen megavolts per metre, and is defined by the maximum electric field that can exist between metallic components in a cavity.
“Nobody knows exactly what’s happening at the [metallic] surface and this is still an active field of research…but when the fields get too large something like tiny little pyramids grow on the surface, and then electrons spray out and the field just breaks down,” says Peter Hommelhoff of Friedrich-Alexander University Erlangen-Nürnberg in Germany.
The cost and technological challenges of conventional accelerators mean that researchers are keen on developing alternative acceleration methods. In this latest research, the oscillating electric fields are created by firing laser pulses into tiny optical cavities made from silicon nanostructures.
Hommelhoff says it took almost thirty years before physicists realized that electron acceleration could also be achieved using nanophotonic cavities driven by optical-frequency light. Using optical light helps scale down the device because the wavelength of the radiation is much shorter than that of microwaves.
No metal required
Hommelhoff points out another important benefit of this approach: “When you drive these frequencies with laser light, you don’t need metal structures”. He adds, “It suffices if you just use regular glass…and you can generate the same mode that you can generate with microwave cavities and microwave fields”.
As the cavity is an insulator, high concentrations of charge do not appear at points on the surface. As a result, the only limit to the acceleration gradient is the electrical breakdown field of the material.
In principle, this allows for the nanophotonic integration of a particle accelerator, producing bunches of electrons in a tiny, precisely-focused beamline. However, there are practical challenges. The electrons in each bunch repel each other and holding a bunch together requires focusing by external forces. Moreover, compression of a bunch in one direction causes it to spread in other directions.
Repulsion problem
In previous work, researchers including Hommelhoff and Olav Solgaard of Stanford University in California have demonstrated that this repulsion problem could be mitigated using alternating phase focusing. In this technique, electrons are alternately confined in one direction and then the other, producing an oscillating field distribution.
Now, new work on these accelerators has been done by two independent research groups. One was led by Hommelhoff at Friedrich-Alexander University. The other group was a collaboration between Stanford scientists led by Solgaard and researchers at TU Darmstadt in Germany led by Uwe Niedermayer. Both teams created nanophotonic dielectric laser accelerators that boosted the energy of electron bunches without the bunches breaking up. Solgaard and Niedermeyer’s team fabricated two accelerators – one designed at Stanford and one at TU Darmstadt. One accelerator boosted the energy of 96 keV electrons by 25% over a distance of just 708 μm. This is about ten times the thickness of a human hair.
“I think that I have put more force on an electron than anybody else ever,” says Solgaard.
The Hommelhoff group’s device worked at lower energies, accelerating electrons from 28.4 keV to 40.7 keV over 500 μm. This presented its own challenges, as Hommelhoff explains. “When you want to accelerate electrons that are non-relativistic – in our case they only travel with one third of the speed of light – it’s not so easy and it’s less efficient to generate the optical mode that co-propagates with the electrons.”
Higher breakdown fields
The researchers are now looking to achieve even higher field gradients by fabricating devices in materials with higher breakdown fields than silicon. They believe that in the near term their acceleration schemes could find applications in medical imaging and in searches for dark matter.

New particle accelerator is driven by curved laser beams
Solgaard says he “might be in a very small minority thinking this is going to play a role in high-energy physics,” but that the technology should be usable in materials such as quartz, whose breakdown field is almost 1000 times that of a traditional accelerator. “Our millimetre becomes a metre,” he says; “by the time we get to a metre we should match SLAC in energy…Think about having an accelerator sitting in my office that matches SLAC.”
“I think these [two teams] have demonstrated an important new step towards a real accelerator on a chip,” says accelerator scientist Carsten Welsch of the University of Liverpool in the UK. However, he cautions that much remains to be done in terms of beam control and miniature diagnostics. In terms of applications, he says: “I share their optimism for catheter-like medical applications, bringing electrons to where they are needed, and in particular for mini-light sources where personally I see the biggest potential. The combination of a high quality electron beam and light could really open completely new research opportunities and applications.”
However, Welsch remains unconvinced about applications such as particle colliders, pointing to the required high luminosity and high beam quality needed in such machines. “The next Large Hadron Collider will not be a dielectric laser accelerator,” he concludes.
Hommelhoff and colleagues describe their work in Nature. Solgaard, Niedermeyer and colleagues describe their work on arXiv.