
Glioblastoma (GBM) is the deadliest and most aggressive form of brain cancer. Almost all tumours recur after treatment, as surviving cells transform into more resilient forms over time to resist further therapies. To address this challenge, scientists at the University of Leeds have designed a novel double-barrel nanopipette and used it to investigate the trajectories of individual living GBM cells as they change in response to treatment.
The nanopipette consists of two nanoscopic needles that can simultaneously inject exogenous molecules into and extract cytoplasm samples from a cell. The nanopipette is integrated into a scanning ion conductance microscope (SICM) to perform nanobiopsies of living cells in culture. Unlike existing techniques for studying single cells, which usually destroy the cell, the nanopipette can take repeated biopsies of a living cell without killing it, enabling longitudinal studies of an individual cell’s behaviour over time.
Writing in Science Advances, the researchers explain that SICM works by measuring the ion current between an electrode inserted in a glass nanopipette and a reference electrode immersed in an electrolytic solution containing the cells. Nanobiopsy is performed when an ion current flows through the nanopore at the tip of the nanopipette after applying a voltage between the two electrodes. In their double-barrel nanopipette, one barrel acts as an electrochemical syringe to perform cytoplasmic extractions; the second contains an aqueous electrolyte solution that provides a stable ion current for precise positioning and nanoinjection prior to nanobiopsy.
The semi-automated platform enables extraction of femtolitre volumes of cytoplasm and simultaneous injection into individual cells. The platform provides automated positioning of the nanopipette using feedback control (the ion current drops when the nanopipette approaches the sample), while detection of particular current signatures indicates successful membrane penetration of a single cell.
Longitudinal studies
As a proof-of-concept of the platform’s ability, the researchers conducted longitudinal nanobiopsy of a GBM cell (and its progeny), profiling gene expression changes over 72 h. They performed nanobiopsy prior to therapy, during treatment with radiotherapy and chemotherapy, and post treatment.
“Our method is robust and reproducible, allowing membrane penetration and nanoinjection across different cell types with distinct mechanical properties,” write co-principal investigators Lucy Stead and Paolo Actis. “The average success rate of nanoinjection is 0.89 ± 0.07. Intracellular mRNA is then extracted.”
The researchers investigated the response of GBM cells to the standard treatment of 2 Gy of radiation and 30 µM of temozolomide. They visually tracked individual cells and their progeny over 72 h, with 98% remaining in the microscope’s field-of-view during this time frame – an important factor when aiming to perform longitudinal analysis.
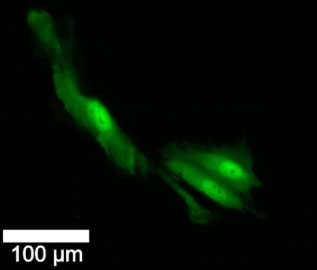
On day 1, the researchers biopsied, injected with a fluorescent dye and imaged each cell. On day 2, half of the cells received irradiation and chemotherapy, while the others served as controls. All cells were imaged on day 2 and 3, and biopsied and injected again on day 4.
In cells that underwent day-1 nanobiopsies, survival was similar between treated and untreated cells, and cell division rates were comparable in the two groups. After 72 h, 63% of untreated control (not biopsied) cells survived, compared with 25% of the treated, biopsied cells. There was no difference in the subsequent death rates of cell subtypes at day 1, irrespective of treatment. However, a much larger proportion of untreated cells switched subtype over time, or produced progeny with a different subtype, than the treated cells.
“This suggests that untreated cells are significantly more plastic over the three-day time course than treated cells,” the researchers write. “The cell phenotype scores of paired day 1 and longitudinal samples revealed that treated cells tend to maintain the same phenotype during therapy, while untreated cells are more likely to switch transcriptional state over 72 h, suggesting that treatment either induces or selects for high transcriptional stability in this established GBM cell line.”
“This is a significant breakthrough,” says Stead. “It is the first time that we have a technology where we can actually monitor the changes taking place after treatment, rather than just assume them. This type of technology is going to provide a layer of understanding that we simply never had before. And that new understanding and insight will lead to new weapons in our armoury against all types of cancer.”
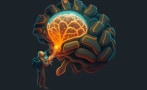
Mechanical nanosurgery attacks aggressive brain cancer
The team is convinced that the ability of these versatile nanoprobes to access the intracellular environment with minimal disruption holds potential to “revolutionize molecular diagnostics, gene and cell therapies”.
“Our future work will focus on increasing the throughput of the technology so that more cells can be analysed,” Actis tells Physics World. “We are working to improve the protocols for analysing the RNA extracted from cells so that more biological information can be gathered. We are also very keen to study more advanced biological models of brain cancer based on patient-derived cells and organoids.”