
Domain walls in graphene form strictly one-dimensional (1D) systems that can become superconducting via the so-called proximity effect. This is the finding of a team led by scientists at the University of Manchester, UK, who uncovered the behaviour by isolating individual domain walls in graphene and studying the transport of electrons within them – something that had never been done before. The discovery has applications in metrology and in some types of quantum bits (qubits), though team member Julien Barrier, who is now a postdoctoral researcher at the Institute of Photonic Sciences (ICFO) in Barcelona, Spain, suggests it might also impact other fields.
“Such strict 1D systems are extremely rare,” Barrier says, “and could serve a number of potential applications.”
The researchers made their 1D system by stacking two layers of graphene (a sheet of carbon just one atom thick) atop each other. When they misalign the layers ever so slightly (less than 0.1°) with respect to each other, the material experiences a strain that makes the atoms in its lattice rearrange themselves into micrometre-scale domains of aligned bilayer graphene.
The narrow regions at the intersection between these domains are known as domain walls, and previous work by members of the same team showed that these walls are very good at conducting electricity. The boundaries between domains were also known (thanks to work by Vladimir Fal’ko and colleagues at Manchester’s National Graphene Institute) to contain special counterpropagating electronic channels that form by hybridizing “edge states” within the conducting domain walls.
These edge states are a consequence of the quantum Hall effect, which occurs when a current passing along the length of a thin conducting sheet gives rise to an extremely precise voltage across opposite surfaces of the sheet. This voltage only occurs when a strong magnetic field is applied perpendicular to the sheet, and it is quantized – that is, it can only change in discrete steps.
One important property of edge states is that electrons in them are said to be “topologically protected” because they can only travel in one direction. They also steer around imperfections or defects in the material without backscattering. Since backscattering is the main energy-dissipating process in electronic devices, such protected states could be useful components in next-generation energy-efficient devices.
Achieving superconductivity in the quantum Hall regime
Over the years, much effort has therefore gone into trying to achieve superconductivity in the quantum Hall regime, as this would mean that the transport of Cooper pairs of electrons (that is, those that travel unhindered through a material to form supercurrents) is mediated by these 1D edge states.
To identify the domain walls in their bilayer graphene sample, the Manchester team turned to a technique called near-field photocurrent imaging developed by Krishna Kumar’s group at the ICFO. This technique provided the information the scientists needed to isolate the domain walls and place them between two superconductors.
They found that doing so not only induces robust superconductivity inside the walls, it also allows the walls to carry individual electronic modes. “This is proof of the 1D nature of this system,” Barrier says.
One unexpected finding is that the superconductivity stems from the proximity of edge states in the domain walls and, in particular, from the strictly 1D states existing within the walls. This means it does not arise from quantum Hall edges on each of the domain walls, as the researchers previously thought.
New approach overcomes previous limitations
The researchers led by Barrier, Na Xin and Andre Geim began their study using a conventional approach in which counterpropagating quantum Hall edge states were brought close together, but these experiments did not produce the results they expected. In previous studies, experimental progress in this direction was limited to observing oscillatory behaviour in the normal (non-superconducting) state, or more recently, to very small supercurrent (of less than 1 nA) at ultralow temperatures of less than 10 mK, Barrier explains.
The new approach, which the team describes in Nature, overcomes these limitations. Thanks to support on the theory side from Fal’ko’s group, the researchers realized that the strictly 1D electronic states they observed in the graphene domain walls hybridize much better with superconductivity than quantum Hall edge states. This realization enabled the researchers to measure supercurrents of a few 10nA at temperatures of ~1K.
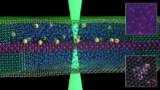
Single atoms swim inside a graphene sandwich
Barrier says the new system could develop along numerous research directions. There is currently an intense interest in quasi-1D (multimode) proximity superconductivity using nanowires, quantum point contacts, quantum dots and other such structures. Indeed, electronic devices containing these structures are already on the market. The new system provides superconductivity via single-mode 1D states and could make such research redundant, he says.
“Our estimation is that electrons propagate in two opposite directions, less than a nanometre apart, without scattering,” he tells Physics World. “Such ballistic 1D systems are exceptionally rare and can be controlled by a gate voltage (like quantum point contacts) and exhibit standing waves (like superconducting quantum dots).”