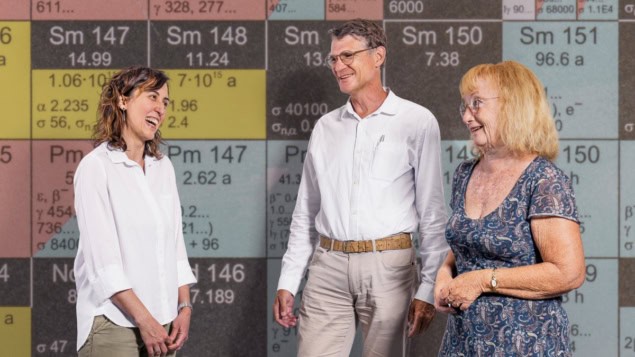
The radioactive half-life of samarium-146 has been measured to the highest accuracy and precision so far. Researchers at the Paul Scherrer Institute (PSI) in Switzerland and the Australian National University in Canberra made their measurement using waste from the PSI’s neutron source and the result should help scientists gain a better understanding of the history of the solar system.
With a half-life of 92 million years, samarium-146 is ideally suited for dating events that occurred early in the history of the solar system. These include volcanic activity on the Moon, the formation of meteorites, and the differentiation of Earth’s interior into distinct layers.
Samarium-146 in the early solar system was probably produced in a nearby supernova as our galaxy was forming about 4.5 billion years ago. Thanks to the isotope’s relatively long half-life, it would have been incorporated into nascent planets and asteroids. The isotope then slowly vanished from the solar system. It is now so rare that it is considered an extinct isotope, whose previous existence is inferred from the presence of the neodymium isotope to which it decays.
There is another isotope, samarium-147, with a half-life that is 1000 times longer than samarium-146. While the two isotopes have identical chemical properties, samarium-147 currently accounts for about 15% of samarium on Earth. Together, these two isotopes can be used for dating rocks, but only if their half-lives are known to sufficiently high accuracy.
Huge range
Unfortunately, the half-life of samarium-146 has proven notoriously difficult to measure. Over the past few decades, numerous studies have placed its value somewhere between 60 and 100 million years, but its exact value within this range has remained uncertain. The main reason for this uncertainty is that the isotope does not occur naturally on Earth and instead is made in tiny quantities in nuclear physics experiments.
In previous studies, the isotope was created by irradiating other samarium isotopes with protons or neutrons. However, this approach has drawbacks. “The main disadvantages are the cost and time required for dedicated irradiation and the fact that the desired isotope is made of the same element as the target material itself,” explains Rugard Dressler at PSI’s Laboratory for Radiochemistry. “This rules out the possibility of separating samarium-146 by chemical means alone.”
To overcome these limitations, a team led by Dorothea Schumann at PSI looked to the Swiss Spallation Neutron Source (SINQ) as a source of the isotope. SINQ creates neutrons by smashing protons into solid targets, which are damaged in the process. To better understand how this damage occurs, a range of different target materials have been irradiated at SINQ. This included tantalum, which Schumann identified as the most promising material to extract a quantity of samarium-146 in solution using a sequence of highly selective radiochemical separation and purification steps.
“Only in this way it was possible to obtain a sufficient amount of samarium-146 for the precise determination of its half-life – a possibility that is not available anywhere else around the world,” explains PSI’s Zeynep Talip.
Then they used some of the solution to create a thin layer of samarium oxide on a graphite substrate. Using mass spectrometers at PSI and in Australia to study their original solution, the team determined that there were 6.28×1013 samarium-146 nuclei in their sample.
Alpha particles
The sample was place at a well-defined distance from a carefully calibrated alpha radiation detector. By measuring the energy of emitted alpha particles, the team confirmed that the particles were produced by the decay of samarium-146. Over the course of three months, they measured the isotope’s decay rate and found it to be just under 54 decays per hour.
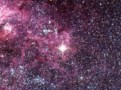
Did a supernova trigger the late Devonian extinction?
From this, they calculated the samarium-146 half-life to be 92 million years, with an uncertainty of just 2.6 million years.
“The half-life derived in our study shows that the results from the last century are compatible with our value within their uncertainties,” Dressler notes. “Furthermore, we were able to reduce the uncertainty considerably.”
This result marks an important breakthrough in an experimental challenge that has persisted for decades, and could soon provide a new window into the distant past. “A more precise determination of the half-life of will pave the way for a more detailed and accurate chronology of processes in our solar system and geological events on Earth,” says Dressler.
The research is described in Scientific Reports.