Particle physicist Juan Pedro Ochoa-Ricoux talks to Katherine Skipper about how the next generation of neutrino experiments will test the boundaries of the Standard Model

It was a once-in-a-lifetime moment during a meeting in 2011 when Juan Pedro Ochoa-Ricoux realized that new physics was emerging in front of his eyes. He was a postdoc at the Lawrence Berkeley National Laboratory in the US, working on the Daya Bay Reactor Neutrino Experiment in China. The team was looking at their first results when they realized that some of their antineutrinos were missing.
Ochoa-Ricoux has been searching for the secrets of neutrinos since he began his master’s degree at the California Institute of Technology (Caltech) in the US in 2003. He then completed his PhD, also at Caltech, in 2009, and is now a professor at the University of California Irvine, where neutrinos are still the focus of his research.
The neutrino’s non-zero mass directly conflicts with the Standard Model of particle physics, which is exciting news for particle physicists like Ochoa-Ricoux. “We actually like it when the theory doesn’t match the experiment,” he jokes, adding that his motivation for studying these elusive particles is for the new physics they could reveal. “We need to know how to extend [the Standard Model] and neutrinos are one area where we know it has to be extended.”
Because they rarely interact with matter, neutrinos are notoriously hard to study. Electron antineutrinos are however produced in measurable quantities by nuclear reactors and this is what Daya Bay was measuring. The experiment consisted of eight detectors measuring the electron antineutrino flux at different distances from six nuclear reactors. As the antineutrinos disperse, the detectors further away are expected to measure a smaller signal than those close by.
However, when Ochoa-Ricoux and his team analysed their results, they found “a deficit in the far location that could not only be explained by the fact that those detectors were farther away”. Neutrinos come in three types, or “flavours”, and it seemed that some of the electron antineutrinos produced in the power plants were changing into tau and muon antineutrinos, meaning the detector didn’t pick them up.
This transformation of neutrino type, also known as “oscillation”, occurs for both neutrinos and antineutrinos. It was first observed in 1998, with the discovery leading to the award of the 2015 Nobel Prize for Physics. However, physicists are still not sure if antineutrinos and neutrinos oscillate in the same way. If they don’t, that could explain why there is more matter than antimatter in the universe.
The mathematics of neutrino oscillation is complex. Among many parameters, physicists define an angle called θ13, which plays a role in determining the probability of certain flavour oscillations. For differences in oscillation probabilities between neutrinos and antineutrinos to be possible, this quantity must be non-zero. When Ochoa-Ricoux was working on the Main Injector Neutrino Oscillation Search (MINOS) at Fermilab in the US for his PhD, he had found tantalizing but inconclusive evidence that θ13 is different from zero.
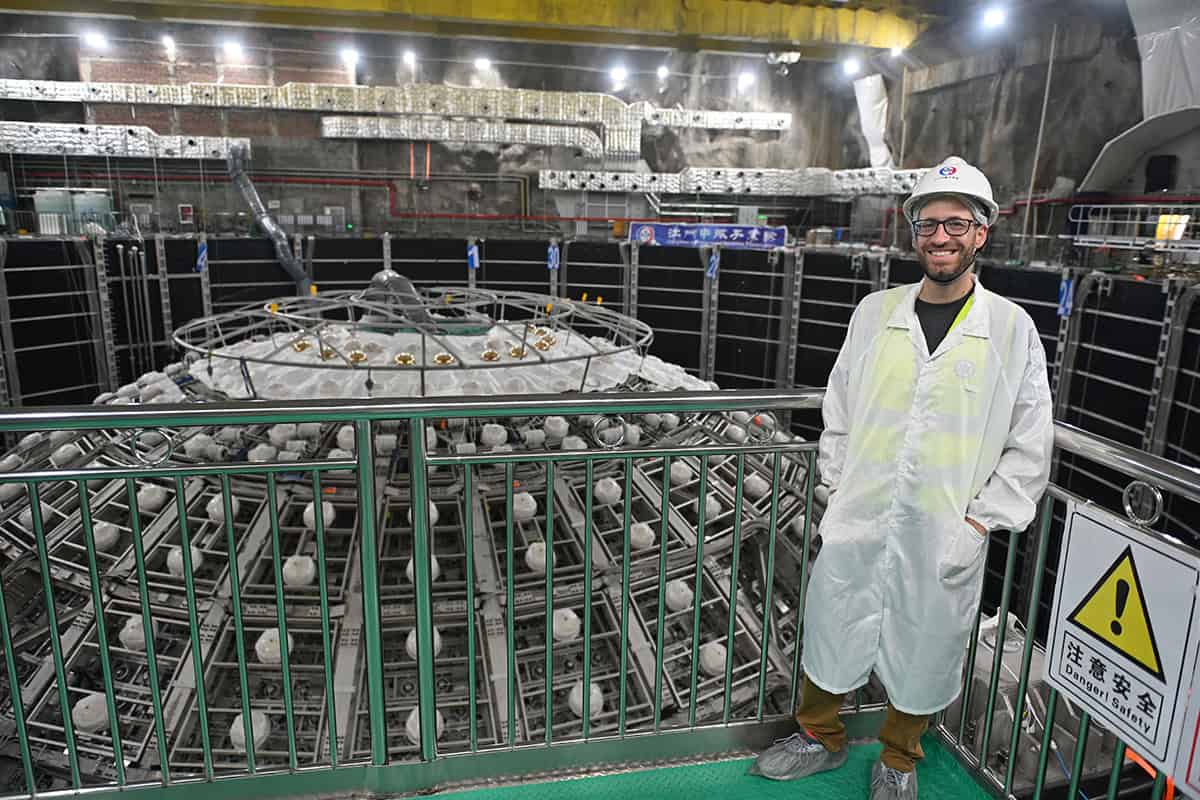
The memorable meeting Ochoa-Ricoux recalled at the start of this article was, however, the first moment he realized “Oh, this is real”. Their antineutrino deficit data eventually proved that the angle is about nine degrees. This discovery set the stage for Ochoa-Ricoux’s career, which, a little like the oscillating neutrino, he describes as a “mixture of everything”.
The asymmetry between antimatter and matter is one of the biggest mysteries in physics and in the next four years, two experiments – HyperKamiokande in Japan and the Deep Underground Neutrino Experiment (DUNE) in the US – will start looking for evidence of matter–antimatter asymmetry in neutrino oscillation (Ochoa-Ricoux is a member of DUNE). “Had θ13 been zero” he says, “my job and my life would have been very very different”.
All hands on deck
On the one hand you analyse the data, but before you can do that, you actually have to build the apparatus
Ochoa-Ricoux wasn’t just analysing the results from Daya Bay, he was also assembling and testing the experiment. This was sometimes frustrating work – he remembers having to painstakingly remeasure detector components because they wouldn’t fit inside the machine. But he emphasizes that this was an important part of the Daya Bay discovery. “On the one hand you analyse the data, but before you can do that, you actually have to build the apparatus,” he says.
While Ochoa-Ricoux now spends much less time climbing inside detector equipment, he is actively involved in designing the next generation of neutrino experiments. As well as DUNE, he works on Daya Bay’s successor, the Jiangmen Underground Neutrino Observatory (JUNO) in China, a nuclear reactor experiment that is projected to start taking data at the end of the year.
The first neutrino oscillation measurement was made in 1998 by the Japanese researcher Takaaki Kajita, who would later share the 2015 Nobel Prize for Physics for his work. However, the experiment where Kajita made this observation, called SuperKamiokande, was originally designed to search for proton decay.
Ochoa-Ricoux thinks that DUNE and JUNO need to be open to finding something equally unexpected. JUNO’s main aim is to determine which neutrino mass is the heaviest by measuring oscillating antineutrinos from nuclear power plants. It will also detect neutrinos coming from the Sun or the atmosphere, and Ochoa-Ricoux thinks this flexibility is vital.
“Sometimes nature will surprise us and we need to be ready for that,” he says, “I think we need to design our experiments in such a way that we can be sensitive to those surprises.”
Exploring the unknown
Experiments like DUNE and JUNO could change our understanding of the universe, but there is no guarantee that neutrinos hold the key to mysteries like matter–antimatter asymmetry. There’s therefore pressure to deliver results, but Ochoa-Ricoux is excited that the field is taking leaps into the unknown.
When you understand your world better, sometimes it’s impossible to predict what applications will come
He also argues that as well as advancing fundamental science, these projects could lead to new technologies. Medical imaging devices like MRI and PET scanners are offshoots of particle physics and he believes that “When you understand your world better, sometimes it’s impossible to predict what applications will come.”
However, at the heart of Ochoa-Ricoux’s mindset is the same fascination with the mysteries of the universe that motivated him to pursue neutrino physics as a student. For him, projects like JUNO and DUNE can justify themselves on those grounds alone. “We’re humans. We need to understand the world we live in. I think that’s highly valuable.”