If you want to see an aurora, you could go to the Arctic. Or you could go to Saturn. Sarah Badman explains how observations of auroras on other planets are revealing new facts about these fascinating and beautiful phenomena
Seeing an aurora such as the “northern lights” is high on many people’s bucket lists. These flickering lights – which appear when charged particles get trapped by the Earth’s magnetic field and crash into the atmosphere, making it glow – are among nature’s most fantastic displays, and there is a lot of luck involved in catching a glimpse of them. You have to be in the right place: typically between 20 and 30 degrees from the pole. You need clear, dark skies, far away from cities and their associated light pollution. And, perhaps most importantly, you need the right “space weather”: a high-speed “solar wind” and a strongly southward interplanetary magnetic field.
One thing you do not need, though, is the Earth itself. With its thick atmosphere and strong magnetic field, our planet is well equipped to give us a good light show, but it is not the only place in our solar system where auroras can happen. Two other examples are Jupiter and Saturn, which have very thick atmospheres (they are the gas giants after all) and strong magnetic fields. Missions to study their auroras are currently under way, giving us more information about these planets’ atmospheres and their surrounding space environments – and producing a few surprises, too.
An aurora’s fingerprint
Here on Earth, and in most other places in the solar system, the Sun is the main source of aurora-producing charged particles. The hot, uppermost layer of the Sun’s atmosphere is the source of the solar wind, a diffuse plasma of electrons and protons travelling through space at about 400 km/s, carrying the Sun’s magnetic field with it. When this solar wind reaches a planet’s magnetic field, it usually deflects around it. However, when the Sun’s magnetic field and that of the planet are anti-parallel to each other, they can merge. When this happens, solar-wind particles are able to enter the planet’s magnetosphere. Some particles then travel directly down into the atmosphere on the planet’s “dayside” (the side facing the Sun) and trigger auroral emission there.
1 Forecasting an aurora
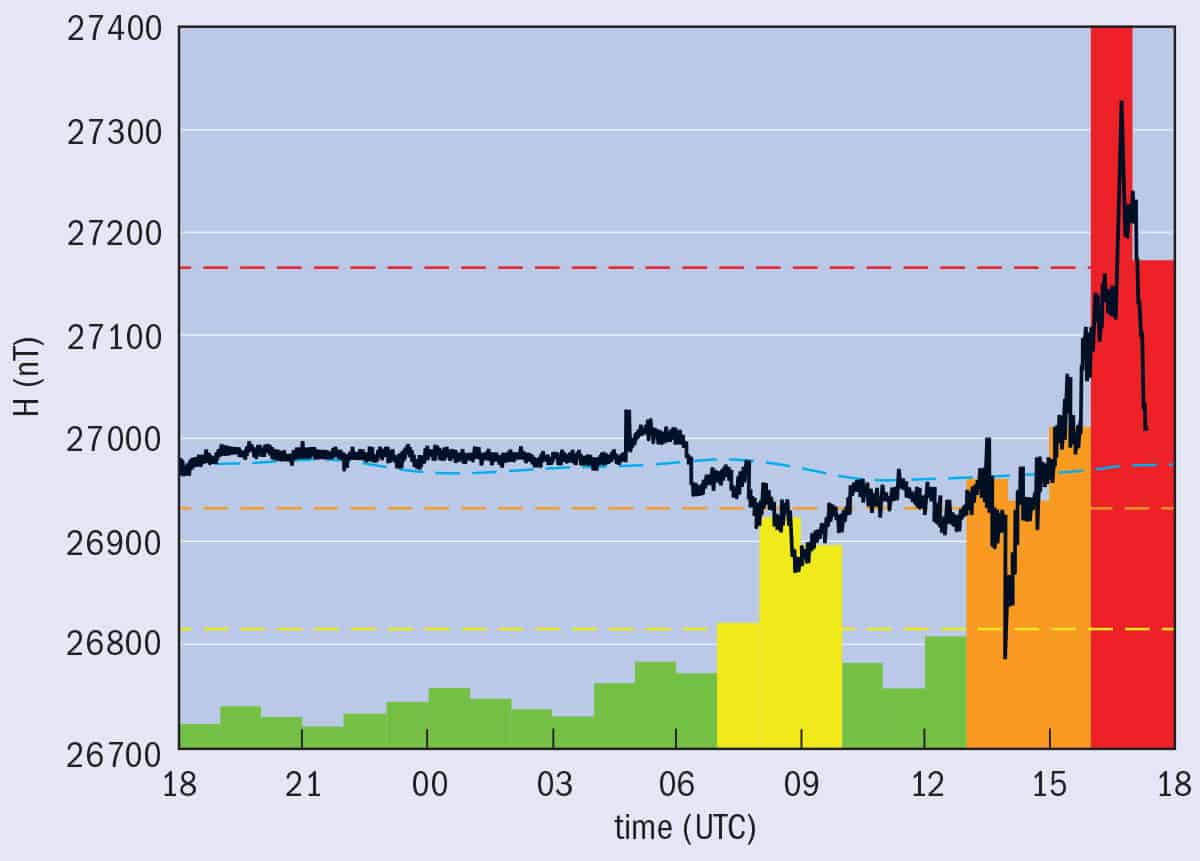
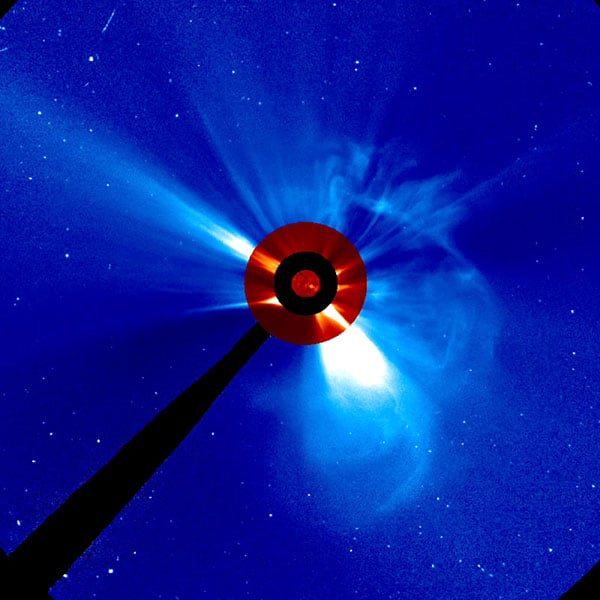
The graph (a) shows the H-component of the magnetic field (the component that points towards magnetic north) as recorded by AuroraWatchUK on 16–17 March 2015. Coloured bars indicate how much the magnetic field at a given moment deviates from the average for a “quiet” day (blue dashed line), with green indicating “quiet”, yellow “minor geomagnetic activity”, amber “active” and red “stormy”. The dashed red line represents the threshold that triggers a “red alert”. The image left (b) shows the coronal mass ejection that caused the 17 March aurora.
But the solar wind also causes the planet’s magnetic field lines to be convected away from the Sun, forming a kind of magnetic “tail”. After an interval in which this magnetic tail becomes “loaded” with field lines, the magnetic field undergoes an explosive reconfiguration and injects plasma back towards the planet on the nightside. Some of this plasma reaches the atmosphere and causes bright auroral displays. Larger events can be triggered by solar activity such as high-speed streams of solar wind or coronal mass ejections (large clouds of solar plasma threaded with magnetic field). When one of these reaches the Earth’s magnetosphere it can transfer a lot of energy, leading to exceptionally large, bright auroras (see figure 1).
Auroras normally form a circular shape around the pole connected to a ring of magnetic field lines. By tracing along these magnetic field lines away from the planet, we can find out where in the magnetosphere the charged particles are coming from. Even something as basic as an aurora’s colour gives us a wealth of information. Because different species of atoms have different energy levels, the jumps between levels determine the energy and hence the wavelength of the light they emit. For example, the common green colour in the northern lights comes from oxygen atoms more than 100 km above the Earth’s surface. Red auroras can be seen when enough electrons excite different transitions in the less dense oxygen higher up in the atmosphere, while a deep red at the bottom of the green curtain is indicative of higher-energy electrons penetrating deeper into the atmosphere and exciting nitrogen molecules.
Flickering lights, alien skies
Extraterrestrial auroras were first detected in 1979 by the Voyager spacecraft at Jupiter and by Pioneer 11 at Saturn. Since the Pioneer and Voyager flybys, auroras have been observed using Earth-based telescopes and the Hubble Space Telescope. Beginning in 2004, though, Saturn’s aurora has been studied extensively by the Cassini spacecraft, which began orbiting the ringed planet that year. Ulyana Dyudina and colleagues at the California Institute of Technology, US, used images from Cassini’s Imaging Science System to show that Saturn’s auroras range in colour from red at the lowest altitudes, to pink slightly higher in the atmosphere, where the emission is brightest, up to a fainter, purple colour at the atmosphere’s outer edge. These colours are characteristic of the hydrogen gas that makes up Saturn’s atmosphere. Further investigations may reveal which specific atomic transitions could emit photons with the observed intensity, helping us understand the density of Saturn’s atmosphere and the range of electron energies that are impacting it.
The shape of Saturn’s aurora is at first glance very similar to that of the Earth. Typically, it forms an oval around the gas giant’s northern and southern magnetic poles. However, there are some differences. Saturn rotates with a period of less than 11 hours and many features in the aurora rotate with the planet’s atmosphere, either at the same rate or some fraction of it. One effect of the rotation is that Saturn’s aurora is usually brighter on the “dawnside” of the planet, where the atmosphere and magnetic field are rotating towards the Sun, compared with the “duskside” where they are rotating away.

Another difference between Earth’s aurora and Saturn’s is the source of the charged particles that cause the atmospheric emission. One of the major discoveries of the Cassini mission has been the presence of geysers on Enceladus, one of Saturn’s moons, and this discovery was actually sparked by observations of Saturn’s magnetic field. The Cassini magnetometer team, led by Michele Dougherty at Imperial College London, UK, identified that Enceladus must be producing plasma because Saturn’s magnetic field lines were perturbed around the moon’s southern pole. Subsequent flybys revealed a series of stripes in the icy surface, from which water plumes are emitted. These water plumes turn out to be a significant source of plasma in Saturn’s neighbourhood.
Most of the plasma originating from Enceladus spreads out into an extended disc and, along with plasma originating from Saturn’s rings and its other icy moons, forms a reservoir for generating Saturn’s auroral oval. This isn’t the only thing going on, though. Observations made while Cassini was approaching Saturn in 2004 revealed that the aurora also responds to the solar wind conditions. When the solar wind blows quickly past the planet and compresses its magnetosphere, then – a bit like what happens on Earth – bright and broad auroral storms occur. Under these conditions, Saturn’s aurora forms a spiral shape: it is broader and located at higher latitude on the nightside of the planet, and curves round through the dayside to a narrower and lower latitude arc near midnight. This indicates that the strongest injection of electrons happens on the nightside and that field lines further from the planet (with footprints at higher latitudes in the atmosphere) become active. All the observations made so far indicate that Saturn’s aurora results from a complex interaction affected by the planet’s rotation, material coming from Enceladus and the surrounding solar wind.
Another effect of Enceladus’ activity is that, as it perturbs the local magnetic field, some of the surrounding plasma is beamed along the magnetic field lines and deposited in Saturn’s atmosphere, producing an auroral spot. Wayne Pryor of Central Arizona College and Abi Rymer from Johns Hopkins University, both in the US, showed that the auroral spot and electron beam were only sometimes present to mark the footprint of Enceladus. The reason for the variability of Enceladus’ auroral spot is still unknown, but could relate to the plume activity on the moon itself.
The green glow of home
The auroras at Saturn will soon be probed in more detail than ever, as Cassini performs the final stages of its mission by going into a highly inclined orbit that passes close to Saturn’s atmosphere, inside its famous rings. Meanwhile, NASA’s Juno mission is scheduled to go into a similarly inclined, low-altitude orbit around Jupiter (see “Brave new Jupiter” by Stephen Ornes). Both missions will measure the energy and direction of charged particles close to their respective planets’ atmosphere and image the aurora at unprecedented spatial resolution. Another exciting opportunity to learn what is behind different auroral features is Jupiter’s largest moon Ganymede, which has its own magnetic field embedded within Jupiter’s, and auroral ovals in its oxygen atmosphere. This system will be studied for the first time by the European Space Agency’s upcoming JUICE mission, due to arrive at Jupiter in 2030.
The best opportunity for humans to see extraterrestrial aurora first-hand would probably come during a mission to Mars
Beyond Saturn in the outer reaches of the solar system, we know (thanks to the Voyager spacecraft flybys in the 1980s) that the ice giants Uranus and Neptune also have auroras. However, it has proven difficult to detect them using Earth-based telescopes, and hence their characteristics are still not understood.
The best opportunity for humans to see extraterrestrial aurora first-hand would probably come during a mission to Mars. Although Mars lacks a global magnetic field like the Earth’s, it does have localized “mushrooms” of magnetic field looping out from the planet’s crust, and Martian auroras have been detected by spacecraft. Observations made by the MAVEN mission, analysed by Nick Schneider of the University of Colorado, US, showed that these Martian auroras are not limited to the regions where the crustal magnetic field is strongest, but are widespread, as the magnetic field carried in the solar wind drapes through the atmosphere. The small amounts of oxygen in Mars’ atmosphere could even give its auroras a familiar green glow – a welcome sight, perhaps, for any homesick observers on the surface of the red planet.