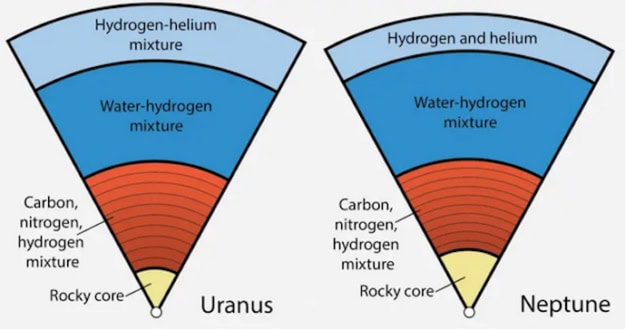
When the Voyager 2 spacecraft flew past Uranus and Neptune in 1986 and 1989, it detected something strange: neither of these “ice giant” planets has a well-defined north and south magnetic pole. This absence has remained mysterious ever since, but simulations performed at the University of California, Berkeley (UCB) in the US have now suggested an explanation. According to UCB planetary scientist Burkhard Militzer, the disorganized magnetic fields of Uranus and Neptune may arise from a separation of the icy fluids that make up their interiors. The theory could be tested in laboratory experiments of fluids at high pressures, as well as by a proposed mission to Uranus in the 2040s.
On Earth, the dipole magnetic field that loops from the North Pole to the South Pole arises from convection in the planet’s liquid-iron outer core. Since Uranus and Neptune lack such a dipole field, this implies that the convective movement of material in their interiors must be very different.
In 2004, planetary scientists Sabine Stanley and Jeremy Bloxham suggested that the planets’ interiors might contain immiscible layers. This separation would make widespread convection impossible, preventing a global dipolar magnetic field from forming, while convection in just one layer would produce the disorganized magnetic field that Voyager 2 observed. However, the nature of these non-mixing layers was still unexplained – hampered, in part, by a lack of data.
“Since both planets have been visited by only one spacecraft (Voyager 2), we do not have many measurements to analyse,” Militzer says.
Two immiscible fluids
To investigate conditions deep beneath Uranus and Neptune’s icy surfaces, Militzer developed computer models to simulate how a mixture of water, methane and ammonia will behave at the temperatures (above 4750 K) and pressures (above 3 x 106 atmospheres) that prevail there. The results surprised him. “One morning, I opened my laptop,” he recalls. “When I started analysing my latest simulations, I could not believe my eyes. An initially homogeneous mixture of water, methane and ammonia had separated into two distinct layers.”
The upper layer, he explains, is thin, rich in water and convecting, which allows it to generate the disordered magnetic field. The lower layer is magnetically inactive and composed of carbon, nitrogen and hydrogen. “This had never been observed before and I could tell right then that this result might allow us to understand what has been going on in the interiors of Uranus and Neptune,” he says.
A plastic polymer-like- and a water-rich layer
Militzer’s model, which he describes in PNAS, shows that the hydrogen content in the methane-ammonia mixture gradually decreases with depth, transforming into a C-N-H fluid. This C-N-H layer is almost like a plastic polymer, Militzer explains, and cannot support even a disorganized magnetic field – unlike the upper, water-rich layer, which likely convects.
A future mission to Uranus with the right instruments on board could provide observational evidence for this structure, Militzer says. “I would advocate for a Doppler imager so we can detect the planet’s natural oscillation frequencies,” he tells Physics World. Though such instruments are expensive and heavy, he says they are essential to detecting the presence of the predicted two ice layers in Uranus’ interior: “Like one can distinguish between an oboe and a clarinet, these frequencies can tell [us] about a planet’s interior structure.”
A follow-up to Voyager 2 could also reveal how the ice giants’ structures have evolved since they formed 4.5 billion years ago. Initially, their interiors would have contained only a single ice layer, and this layer would have generated a strong dipolar magnetic field with well-defined north and south poles. “Then, at some point, this ice separated into two distinct layers and their magnetic field switched from dipolar to disordered fields that we see today,” Militzer explains.

Superionic ice phases could explain unusual magnetic fields around Uranus and Neptune
Determining when this switch occurred would help us understand not only Uranus and Neptune, but also ice giants orbiting stars other than our Sun. “The most common exoplanets discovered to date are around the same size as Uranus and Neptune, so when we observe the magnetic field of such ‘sub-Neptune’ exoplanets in the future, we might be able to say something about their age,” Militzer says.
In the near term, Militzer hopes that experimentalists will be able to test his theory in extremely-high temperatures and pressure fluid systems that mimic the proportions of elements found on Uranus and Neptune. But his long-term hopes are pinned on a new mission that could detect the predicted layers directly. “While I will have long retired when such a detection might eventually be made, I would be so happy to see it in my lifetime,” he says.