Bose-Einstein and Fermi-Dirac statistics, which are by definition mutually exclusive, have been witnessed in the same apparatus for the first time. This is the claim of physicists in the Netherlands and France, who investigated the atomic "Hanbury Brown-Twiss effect" for two isotopes of helium. The discovery could make direct observations of quantum correlations possible (Nature 445 402).
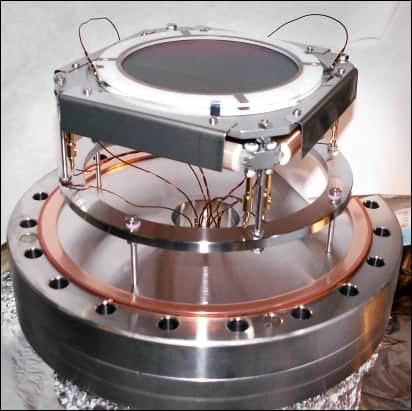
and fermions
Fifty years ago, physicists Robert Hanbury Brown and Richard Twiss noticed that photons from the star Sirius tended to arrive at a pair of detectors at the same time. They realised that this “bunching” effect was permissible for photons because they are bosons, and are therefore inclined to fall into the same quantum state. Since then, the corresponding “antibunching” effect has been noted for fermions, which are governed by the Pauli exclusion principle and so can never occupy the same state.
Now, Chris Westbrook and other physicists from the Université Paris-Sud in France and the Laser Centre Vrije Universiteit in the Netherlands have reported both Hanbury Brown-Twiss (HBT) bunching and antibunching using the same apparatus, which they claim is the first time that Bose-Einstein and Fermi-Dirac statistics (underlying bosons and fermions, respectively) have been directly compared.
In their experiment, the physicists used two ultracold isotopes of helium gas: helium-4 (an innate boson as it has an integer spin number) and helium-3 (an innate fermion as it has a non-integer spin number). In turn, they released the isotopes from a magnetic trap and let them fall under gravity onto a position-sensitive detector. They could then see how well the impacts of the individual atoms were correlated.
Quantum mechanics aside, one might expect the atoms to arrive at the detector randomly. However, Westbrook and colleagues discovered that the helium-4 atoms often arrived together, whereas the helium-3 atoms tended to avoid simultaneous impacts – the tell-tale signs of bosonic bunching and fermionic antibunching.
Westbrook says similar HBT methods could be used to accurately detect quantum correlations in systems where the behaviour is strongly governed by it, such as those displaying the fractional quantum Hall effect. “Being able to observe quantum correlations in such systems would be a very important step forward,” he said. “Analogues to strongly correlated condensed matter systems are being proposed using ultracold atoms.”