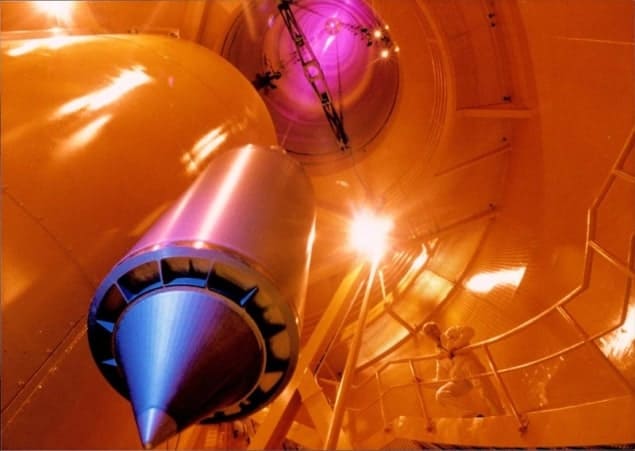
A Bose– Einstein condensate experiment – lasers and all – has been dropped repeatedly from a height of 146 m. Designed by an international team of physicists, the experiment has shown that delicate multiparticle quantum systems can be created and analysed in microgravity environments created during freefall. The result also suggests that it is possible to launch similar experiments into space, where they could test predictions of Einstein’s general theory of relativity.
Bose–Einstein condensates (BECs) are formed when identical atoms with integer spin are cooled until all the atoms are in the same quantum state. This means that a BEC comprising tens of thousands of atoms behaves as a single quantum particle. BECs can be used in matter interferometers, in which a quantum particle is “split” and sent along two different paths before being recombined at a detector – just like a pulse of light in an optical interferometer. Although such experiments have been done with single atoms, their precision is boosted significantly when a BEC is used.
Because BECs are massive objects they are particularly suited to interferometry experiments that measure tiny differences in gravity between two paths. Placing BEC interferometers in microgravity environments such as drop towers or parabolic flights, would allow physicists to test aspects of general relativity to much higher precision than is possible today. These include the geodetic effect and the Lense–Thirring effect, which describe the space-distorting effects of the Earth’s mass and rotation respectively.
Huge technical challenges
However, launching a BEC into space – or even dropping it a few hundred metres – involves huge technical challenges because the BEC must be prepared and maintained in ultrahigh vacuum at ultracold temperatures in a process involving the precise application of magnetic fields and laser light.
Now, Ernst Rasel and colleagues at Leibniz University in Hanover, Germany have built an entire BEC experiment that can be dropped repeatedly from a height of 146 m. The team, which also includes researchers from other universities in Germany, the UK and France, begins with collection of about 10 million cold rubidium-87 atoms. These are loaded into a magneto-optical trap within the drop capsule – a 215cm-long cylinder with a 60 cm radius. The capsule is positioned at the top of the ZARM drop tower in Bremen. When in freefall, gravity inside the capsule can be as little as 10–5 of terrestrial gravity.
The capsule is released and the team allow it to drop for 1 s to allow for the initial vibrations of the capsule to dampen out. The freefall continues as the atoms are further cooled by a laser technique called “optical molasses”, followed by evaporative cooling to create a BEC of about 10,000 atoms at a temperature of about 10 nK.
Expanding BEC
Next, the BEC is released very gently from the magnetic trapping potential, putting it in a state of very slow expansion. This is necessary for long observation times since faster expansion would quickly make the condensate too dilute for imaging – which is done by shining a laser on the BEC and looking at the shadow it casts on a CCD camera.
The team were able to track the motion of the BEC relative to the capsule as the freefall continued for another 1 s. They found that the centre of mass of the BEC moved about 3 mm relative to the capsule over this time. This motion is not of gravitational origin. Instead, the team has shown that most of this effect is caused by tiny residual magnetic fields inside the experiment.
Analysis of the expansion of the BEC also revealed the presence of residual fields, which stretched the BEC along the vertical direction and squeezed it horizontally.
Rasel told physicsworld.com that the team will soon repeat their measurements using a BEC made from atoms in a slightly different quantum state. These are not affected by stray magnetic fields and will therefore lead to a better tool for making gravitational measurements.
Atom interferometer planned
Looking further into the future, the team also plans to build a microgravity atom interferometer in which the atoms are split into two states and then recombined by the absorption and emission of photons. They also plan to create BECs containing two types of atom to see if both behave in the same way.
The research is partially funded by the German Space Agency, and Rasel hopes that it could lead to BECs being studied in space. Such experiments could be used to detect gravitational waves and the comparison of experiments in space and Earth could provide very precise tests of the equivalence principle of general relatively.
Holger Mueller of the University of Calfornia, Berkeley, said that the experiment “represents a very important milestone” in the development of atom interferometers that can be deployed in space. “Space operation requires new technology that is very, very challenging to develop”, he added.
The work is described in Science.