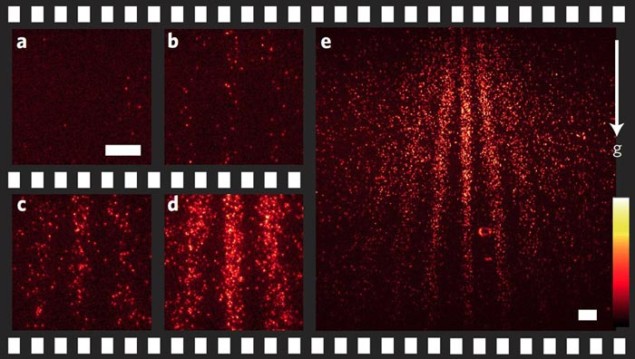
The first real-time movie of large molecules creating an interference pattern after passing through two slits has been made by an international team of physicists. As well as being a beautiful example of the wave–particle duality of quantum mechanics, the technique could provide further insight into the boundaries between quantum and classical physics.
The build-up of an interference pattern as individual particles pass through two side-by-side slits in a screen is one of the most famous examples of how an entity such as an electron can behave both as a particle and a wave. This research has its roots in the famous double-slit experiment carried out by Thomas Young in the early 1800s. When Young shone light through his apparatus, he saw a pattern of bright and dark fringes that could only be explained by the interference of wavefronts. In the 1920s it was shown that the same occurred to electrons, establishing the concept of wave–particle duality. More recently, similar behavior has been seen using molecules containing as many as 400 atoms.
Physicists have also shown that individual particles create an interference pattern that builds up as they pass through the slits one by one and then arrive at a detector. This confirms that each individual particle does indeed behave like a wave as it passes through the slits. Observing this behaviour in large molecules is particularly interesting because it allows researchers to investigate whether there is a threshold at which particles stop behaving like waves and begin to obey the classical laws of physics.
Innovative interference
Now, physicists at institutes in Austria, Israel, Switzerland and Germany have watched in real time as interference patterns were created by 58-atom phthalocyanine molecules (C32H18N8) and 114-atom phthalocyanine derivatives (C48H26F24N8O8) – the latter being the largest ever molecule to be studied in this way. The molecules were produced using micro-evaporation, in which a laser was focused on a thin layer of the compound. This reduced the heat load to the sample, preventing the molecules from decomposing and providing the researchers with an intense and coherent beam of large organic molecules.
The team also created a silicon-nitride diffraction grating with a separation of 100 nm between slits. This ensured that the diffraction angle was large enough to be resolved after the molecules passed through the slits. Furthermore, the grating was just 10 nm thick – around 16 times thinner than previous gratings – in order to reduce interactions between the molecules and the grating material.
Another important innovation was the use of fluorescence microscopy to detect the molecules. This involved exciting the molecules with a laser, and their emitted light was imaged onto an electron-multiplying charge-coupled device (EMCCD) camera. This technique, which allowed each molecule’s position to be determined with an accuracy of 10 nm, was around 10,000 times more sensitive than previous detection methods.
A textbook pattern
The end product is a movie showing the gradual build-up of the quantum interference pattern over 90 min, with each molecule appearing as a fluorescent speck against the dark background.
“The arrival of each single radiating molecule is objectively unpredictable and yet the ensemble reveals the perfect deterministic interference pattern,” says team member Markus Arndt from the University of Vienna. “Previous experiments could see interference but they were not able to store the particles on a detector for future analysis. Fluorescence imaging visualizes the particle nature of the molecules much better than any of the earlier methods and it can do that for hours after the experiment.”
The team used these images to plot 1D diffraction curves, integrating the patterns over a section of the molecules’ velocity distribution. As expected, the curves show a strong central peak, surrounded by weaker secondary peaks – described by the researchers as a “textbook-like diffraction of plane waves at a grating”.
Quantum limits
“Studying quantum interference of large molecules is important because it is a way to explore how far the realm of quantum behaviour can be extended to macroscopic objects,” says Wieland Schöllkopf, a physicist at the Fritz-Haber-Institut der Max-Planck-Gesellschaft in Berlin who was not involved in the study. “I think with ever more ingenious experimental techniques like, for instance, the nanotechnologies used by the Vienna group, it will be possible to push the limits further and further.”
Arndt believes that their technologies can now be scaled up to higher molecular masses. “Quantum mechanics has never been tested for this parameter regime and it is the task of experimentalists to explore the unexplored,” says Arndt. “Whether our world is purely quantum or whether there is a factual transition to classical physics is open to future experiments.”
The research is described in Nature Nanotechnology.
A video of the movie can be viewed here.