Konstantin Batygin explains what led him and astronomer Mike Brown to propose the existence of a ninth planet in our solar system

As one of the oldest forms of natural science, astronomy has enjoyed a long and dramatic history. However, it was not until the early 1600s that the entire discipline was kicked into high gear by Galileo’s adoption of the telescope as a scientific instrument. No longer bound by the resolving power of the human eye, astronomers had finally attained the freedom to search the night skies for the wandering motion of the faintest stars. The door to the discovery of additional planets that orbit the Sun had been cracked open.
In terms of sheer numbers, efforts to expand the solar system’s planetary album have yielded rather unimpressive results. Over the last four centuries, only two planets that were not known to ancient civilizations have been found. The discovery of the first of these planets, Georgium Sidus (now known as Uranus), was announced by William Herschel at the time of the American Revolutionary War, in 1781. This finding simultaneously marked the beginning and the end of purely astronomical detection of planets in the solar system. Indeed, the revelation of the next planet would rely more on celestial mechanics than on a telescope.
Soon after Herschel’s announcement of Uranus, astronomers began to compute its orbital motion and flirt with the idea that an additional, more distant object could gravitationally perturb its trajectory. Among the first astronomers to lead this charge was Anders Johan Lexell. In a set of compiled astronomical tables published in 1821, which included accidental observations of Uranus that predated its formal discovery, Alexis Bouvard (then director of the Observatoire de Paris) noted that Uranus was indeed deviating from its predicted path. Without discounting the possibility of spurious data, Bouvard joined Lexell in speculating that the irregularities in Uranian motion could be caused by an additional planet.
It would take more than two decades before the promise of Bouvard’s data came to fruition. In a parallel set of calculations completed in 1846, John Couch Adams and Urbain Le Verrier independently predicted the existence of Neptune. Although the computed orbital period and mass of the putative Neptune exceeded the real values by a significant margin, the calculations gave the correct location in the sky. Then, in a remarkable feat of observational confirmation of theoretical results, Neptune was spotted by Johann Galle on the first night of his observational campaign later that same year.
Once the ability to deduce the presence of an additional planet using orbital irregularities had been demonstrated, a number of contemporary mathematicians attempted to derive the existence of even more distant objects using existing data. As a result, by the early 1900s there was no shortage of hypothetical planets beyond Neptune. One particularly notable prediction was Percival Lowell’s famed Planet X hypothesis, which led to the accidental discovery of Pluto in 1930 (see “Our new view of Pluto”, July 2016, pp40–43 in print).
In the end, it was unmanned spaceflight that killed Planet X. Following Voyager 2’s 1989 encounter with Neptune, the planet was recognized to be a fraction of a per cent less massive than previously thought. Like a Rubik’s cube snapping into its orderly configuration, this small change cleansed the solar system’s astronomical charts of any irregularities, and erased the theoretical need for Planet X. As history shows, the claims of additional planets following Neptune’s discovery had more to do with erroneous interpretation of the observational data than anything else. Every time the observations seemed to call for the introduction of another planet, further analysis revealed that the apparent anomalies could be fully reconciled within the framework of the known solar system.
The first discoveries of icy debris beyond the orbit of Neptune, now collectively known as the Kuiper belt, conformed to this narrative well. As observational surveys began to expose the intricate dynamical structure of the Kuiper belt, it became increasingly clear that virtually every Kuiper belt object’s orbital evolution could be explained through gravitational interactions with Neptune. While some objects are currently locked into orbital resonances with Neptune, others show signs of having been tethered by its gravitational pull in the past. Hence, at the turn of the 21st century, the large-scale architecture of the solar system showed no signs of abnormality whatsoever.
Kuiper belt clues
The solar system in 2016 tells a very different story. Over the course of the last 15 years, observational mapping of the Kuiper belt has revealed a simple, fundamental fact: the orbital arrangement of the most distant bodies in the Kuiper belt is incompatible with an eight-planet solar system.
The first real hint that the solar system still has some tricks up its sleeve came in 2003, when a team of astronomers led by Mike Brown discovered Sedna, a Kuiper belt object (KBO) unlike any other. Whereas most known KBOs have orbital periods not too different from the approximately 250-year period of Pluto, Sedna requires more than 11,000 years to complete its journey around the Sun. Another impressive feature of Sedna’s orbit is its staggering ellipticity. At its furthest from the Sun, Sedna swings out to almost 1000 astronomical units (where one astronomical unit is the mean Earth–Sun distance, roughly 150 million kilometres).

The truly remarkable thing about Sedna, however, is that its orbit is not elliptical enough. Most KBO orbits appear to physically hug the orbit of Neptune. That is because – due to gravitational potential being conservative – any small object that has been sent on a highly elliptical trajectory by Neptune must come back to its point of origin, i.e. the orbit of Neptune. Sedna’s orbit represented the first true exception to this rule: even at its closest approach to the Sun, Sedna remains more than twice as far away from the Sun as Neptune. As a result, Sedna’s origin posed somewhat of a mystery. A body that never experienced direct interactions with Neptune could not have been placed on its orbit by Neptune alone.
In a paper detailing Sedna’s discovery, Brown, Chad Trujillo and David Rabinowitz speculated on the various scenarios that could potentially account for the genesis of its strange orbit, including a scenario where an undiscovered Earth-mass planet lurks beyond the orbit of Neptune (2004 Astrophys. J. 617 645). Around the same time, Brett Gladman and Collin Chan independently discussed the possibility of a rogue planet shaping some features of the Kuiper belt. A similar viewpoint was adopted by yet another researcher, Rodney Gomes, in Brazil. In some sense, the discussion mirrored the Lexell–Bouvard speculation of the early 1800s, in which close examination of Uranus had given clues to the existence of Neptune. Clear echoes of a distant perturbing body were beginning to emerge.
Sedna’s loneliness as an outlier finally came to an end in 2014, when Trujillo and Scott Sheppard discovered a second Sedna-like object, 2012 VP113 (Nature 507 471). With a perihelion distance (a body’s closest distance to the Sun) even larger than that of Sedna, 2012 VP113 confirmed that these objects are not outliers: they are members of a separate, detached population of KBOs. It was with this very paper in hand, and a facial expression showing a combination of excitement and concern, that Mike Brown walked into my office two years ago.
Gravity of the situation
“Have you seen how weird this is?” Mike asked, pointing to figure 3 in Trujillo and Sheppard’s paper. Here the authors note that all KBOs with orbits with perihelion distances beyond Neptune and with periods longer than 2000 years tend to cluster in their argument of perihelion. (The argument of perihelion is a bizarre parameter: it is the angle between the point at which an orbit intersects the ecliptic plane while travelling from south to north on the sky and the point of closest approach to the Sun. Taken at face value, a collection of similarly inclined orbits that cluster in the argument of perihelion would trace out a cone-like structure.) Not swaying from tradition, Trujillo and Sheppard had speculated that this clustering could be due to an unseen, few-Earth-mass planet, with a circular orbit and a period equal to that of 2012 VP113. However, the authors simultaneously acknowledged that such a planet could not, in fact, explain the data adequately.
Intrigued, Mike and I examined the data ourselves. The clustering pointed out by Trujillo and Sheppard emerged on the computer screen. However, to our surprise, this clustering was not alone – other orbital co-ordinates were grouped as well. Immediately, it was clear that the clustering of the argument of perihelion is only part of the full picture. A closer look at the data showed that six objects that occupy the most expansive orbits in the Kuiper belt (including Sedna and 2012 VP113) trace out elliptical paths that point into approximately the same direction in physical space, and lie in approximately the same plane.
Mike and I were genuinely perplexed. Could the confinement of the orbits be due to an observational bias, or perhaps to mere coincidence? Will any theory aimed at explaining these observations suffer the same fate as Lowell’s Planet X hypothesis (i.e. the need for it disappears once more accurate observations are made)? Thankfully, the probability of the observed alignment being fortuitous can be assessed in a statistically rigorous manner, owing to the large size of the comparison sample (i.e. other KBOs that are found at a similar radial distance to our objects of interest). The probability that the alignment is a fluke clocked in at only 0.007%. Not a great gamble.
Could this orbital alignment be a relic of an encounter with a passing star during the solar system’s infancy? An application of simple mean-field perturbation theory showed that if allowed to evolve under the gravitational influence of Jupiter, Saturn, Uranus and Neptune, these objects’ orbits would become randomly oriented on timescales much shorter than the multi-billion-year lifetime of the solar system. So the dynamical origin of the peculiar structure of the Kuiper belt cannot be outsourced to the distant past – something is holding the orbits together right now.
Having quadruple-checked our results, we sat on my couch and stared silently at each other. The gravity of the situation began to sink in. Could it truly be that after 170 years of false alarms and non-detections, we had stumbled upon actual evidence that the solar system’s planetary catalogue is incomplete? We got to work.
Glimpse of hope
Our progress was initially anything but rapid. Coming from observational and theoretical backgrounds respectively, Mike and I don’t always speak the same language, and would spend hours arguing profusely, only to later realize that we are in fact, saying the exact same thing. Then there were all the calculations that did not pan out. Ideas crowding our outtakes reel range from models where the self-gravity of the Kuiper belt itself keeps the observed structure intact, to a scenario where the orbit of a distant planet cradles the orbits of KBOs from the outside, maintaining the same average orientation. Each hypothesis failed when confronted with the data.
Last summer brought our first glimpse of hope. We were running a series of evolutionary numerical experiments, starting off each time with a randomized disc of planetary building blocks, or “planetesimals”. We placed these objects in eccentric, Neptune-hugging orbits that were allowed to evolve under the gravitational influence of a distant perturber, which we dubbed “Planet Nine”. We began to notice that groups of planetesimals emerged in orbits that were co-linear and spatially confined. Intriguingly, this would occur only if Planet Nine was chosen to be about 10 times more massive than the Earth, and to reside on a highly eccentric orbit. More unexpectedly, the confined orbits would cluster in a configuration where the long axes of their orbits are anti-aligned with respect to Planet Nine.
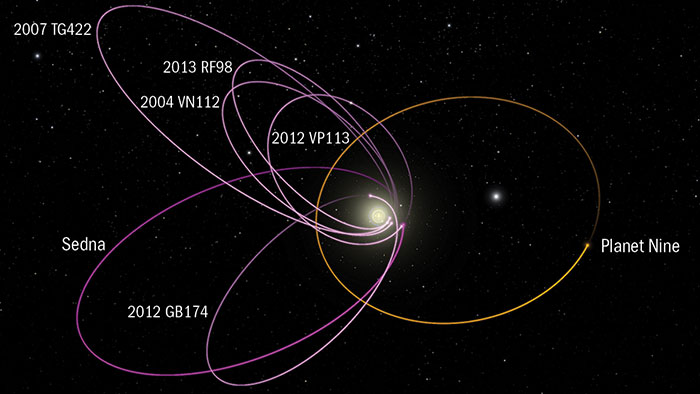
At first glance, this outcome was puzzling. If the trajectories of the KBOs intersect the orbit of the perturbing planet, wouldn’t the objects have been scattered away at some point over the past few billion years? It turns out that the answer can be summarized in one word: resonance. Just as the overlapping orbits of Pluto and Neptune are protected from close encounters by a clockwork-like orbital period ratio of 3:2, the confined orbits of the distant Kuiper belt glean long-term stability from resonances with Planet Nine. However, the latter picture is somewhat more complex: the resonances at play are exotic and interconnected, yielding orbital evolution that is fundamentally chaotic. In other words, perturbed by Planet Nine, the distant orbits of the Kuiper belt remain approximately aligned, while changing their shape unpredictably on million-year timescales.
Surprising and unforeseen results continued to accrue. Upon a cursory examination of the simulation data, we noticed that gravitational torques exerted onto the Kuiper belt by Planet Nine would induce long-period oscillations in the perihelion distances of the confined KBOs. This naturally generated detached orbits, such as those of Sedna and 2012 VP113. Suddenly, the origins of these objects became abundantly clear: they are regular KBOs that have been pulled away from their original locations by Planet Nine. Moreover, the evolutionary calculations suggested that if we were to revisit the Kuiper belt in a hundred million years, objects like Sedna and VP would once again look like conventional, garden-variety KBOs, while some of the more typical objects would now be in detached orbits.
Finally, there was a weird, crazy twist. In every simulation that produced a synthetic Kuiper belt that resembled the real one, the model also consistently generated orbits that were nearly perpendicular to the plane of the solar system. Given that there is virtually no other way to produce such extreme inclinations in the solar system, we thought that this would be a strong prediction: if such objects were ever discovered, they would constitute tangible evidence for the existence of Planet Nine.
Planet Nine falls into place
Caught up in our attempts to understand the dynamics of the simulations, we had forgotten to check the actual data. Then, on a sunny afternoon in October, we plotted the observed catalogue of objects on top of our model’s predictions to see if, by any chance, highly inclined bodies of the type our simulations predicted had been discovered since we last checked. And there they were – five objects, accidentally detected by a near-Earth asteroid survey, exactly where our model predicted them to be. Once again, Mike and I sat in our seats and stared at each other in silence, allowing reality to slowly sink in.
For the first time in our joint scientific journey, we realized that Planet Nine is really out there. The theoretical model did not just explain the peculiar clustering of the orbital angles. It tied together three, seemingly unrelated aspects of the Kuiper belt into a single, unified picture: physical alignment of the distant orbits; generation of detached objects such as Sedna; and the existence of a population tracing out perpendicular orbital trajectories. As far as merits of a dynamical model go, it is difficult to ask for more. However, it is simultaneously important to keep in mind that until Planet Nine is caught on camera, it remains a theoretical prediction.
Fortunately, the prospects of confirming Planet Nine observationally are not as dim as the planet itself. Given our model’s best estimates, Planet Nine has an apparent magnitude of 24–25 and currently lies in the vicinity of Orion’s shield. Detecting its parallactic motion is well within the capabilities of the Subaru Telescope on Mauna Kea in Hawaii, and multiple groups have already set out on the observational hunt. It may take years, but I, for one, am confident that we will one day wake up to learn that solar photons that reflected off Planet Nine’s frigid surface have landed onto the aperture of a terrestrial telescope.
For now, I wait anxiously for that day.