Tucked away in California’s Death Valley National Park, the flat basin known as Racetrack Playa is littered with stones that seem to migrate across the landscape. But how do they do it? Brian Jackson describes how he and collaborators Ralph Lorenz and Richard Norris solved a long-standing mystery
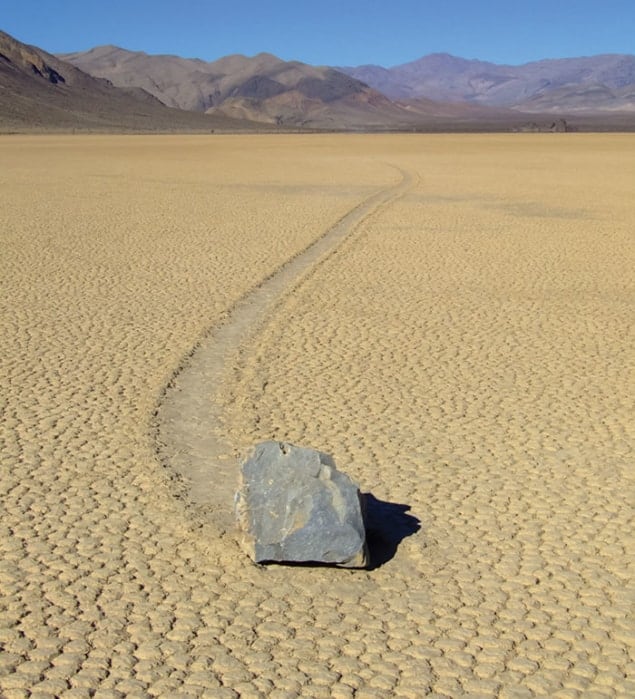
Racetrack’s “sailing stones” range from pebbles to boulders weighing hundreds of kilograms. They originate from the ancient dolomite cliffs at the playa’s southern end, where incessant winter freeze–thaw cycles cause pieces to splinter off and tumble down onto the flat expanse below. The stones’ dark colour and angularity contrast with the playa’s smooth, beige surface, giving the distinct impression of a man-made sculpture. And trailing behind many of the rocks are long, shallow furrows in the hard-packed clay: a telltale sign that, at some point, these stones must have been on the move.
The rock trails wind erratically, sometimes turning 90°, sometimes doubling back on themselves. They often terminate with a rock at one end that has mounded up clay in front of it, like the scrapings of a bulldozer. Some furrows run parallel to one another, wheeling like line-of-battle ships tacking into the wind. Apparently, the stones formed the furrows by ploughing through the clay when it was wet. But how?
This question has plagued generations of visitors to Racetrack Playa, and scientists have tried to answer it for decades. One of the first, John Shelton of Pomona College, landed a small aircraft on the playa back in 1953. He found that the airflow from the plane’s propeller (equivalent to a 70 km/h wind) was enough to flip over one of the rocks, suggesting that wind could play a role in their movement. These days, US federal law prohibits such adventurous experiments on the playa, which is now a protected wilderness area. However, later, less disruptive research by San José State University geologist Paula Messina also found support for the wind theory. During her graduate studies, Messina – known as the mother of modern Racetrack research – measured high and highly variable winds on the playa. In 2000 she and her longtime collaborator (and husband) Phil Stoffer demonstrated that the surrounding valley’s topography channels these winds onto the playa’s southern edge.
Even so, a simple calculation shows that to move a typical sailing stone with a diameter of about 10 cm and a mass of 3 kg, the wind speeds would need to exceed 50 m/s or 180 km/h – much higher than anyone has observed on Racetrack. To circumvent the need for high winds, several alternative theories have been proposed over the decades, from the growth of slippery algal or bacterial mats when the playa is wet to pranking rituals by university students and even the actions of passing aliens. But all these hypotheses, from the plausible to the absurd, remained uncorroborated, and exhaustive surveys of the sailing stones and their trails by Messina during the 1990s and early 2000s produced interesting but ambiguous results that didn’t point towards any one hypothesis.
Solving the mystery of the sailing stones would take years’ more fieldwork, an array of hi-tech instruments, and more than a little luck. On a cold December morning in 2013, all these things came together. As members of our research group stood on the banks of Racetrack, we finally saw the sailing stones in motion – and the mechanism for their wanderings proved more complex and subtle than any of us had suspected.
Remote observations
Racetrack Playa is a closed basin nestled high in the Last Chance Range, more than two hours’ drive from the Death Valley National Park visitor centre. To get there, you head north-west from the centre on Highway 190, then turn off onto a rough gravel road that takes you past the Ubehebe volcanic craters, through a forest of Joshua trees, and down into the desolate Panamint Valley. After you pass the Teakettle Junction signpost (bestrewn with signed teakettles from generations of park visitors), Racetrack Playa appears as a flat, kidney-shaped landform in the hazy distance.
My first visit to Racetrack came during a class field trip when I was in graduate school at the University of Arizona’s Lunar and Planetary Laboratory. My friend and colleague Ralph Lorenz, a research scientist in the lab, came on the trip, too. Stepping onto the windswept playa, we were immediately struck by its austere beauty and gripped by the mystery of the sailing stones, squatting on the surface like desert sphinxes. Soon after the trip, Ralph and I began preparing to return.
The same technology revolution that brought us iPhones and military drones has also provided scientists a wealth of instrumentation for in situ observations of natural phenomena. Ralph had already collected a considerable arsenal of these tools through his other desert fieldwork, and deploying them on Racetrack over the winter to observe meteorological conditions seemed like an obvious thing to do. With luck, we thought, we might even catch the rocks in motion.
After obtaining a research permit from the National Park Service, we began deploying our time-lapse cameras, wind gauges and thermometers in the late autumn of 2007. Sometimes, we set up equipment on nearby playas (such as Little Bonnie Claire, located only an hour from Death Valley) where rock tracks have also been seen, although not as dramatically as on Racetrack. Each autumn, we cast our scientific net in order to observe the rainy season during winter, when the rocks probably moved, and each spring, we hauled in our catch of data. By spring 2012 we’d collected a wealth of data about winter weather on Racetrack. But somehow, in six winters, there had been no significant rock movements. Perhaps the rocks were camera-shy?
Still, we had made some progress. Our data showed that Death Valley and the surrounding region is a meteorologically dynamic place. At more than 1 km elevation, Racetrack’s winters are cold as well as wet, and snow storms are common, often covering the playa in centimetres of snow. In our time-lapse imagery, we watched the winter Sun creep out onto the playa and melt this snow to produce a shallow, crystal-clear pond. We knew beforehand that such ponds were responsible for the playa’s extreme flatness; by periodically wetting and smoothing the surface clay, they keep Racetrack nearly level, with only a few centimetres of relief across its entire 2 km by 4.5 km area. We saw that these ponds persisted for days or weeks at a time, and night-time lows would often re-freeze them, producing large ice floes many tens of metres across.
The data began to implicate ice as the key to solving the sailing-stone enigma, although this wasn’t an entirely original result. In 1955 George Stanley, a geologist at California State University, Fresno, had suggested the moving rocks might be frozen into large ice floes. By physically connecting the rocks to something with a bigger surface area, Stanley reasoned, these ice floes would increase the total wind drag on the rocks and, if they were big enough, act like sails to help them move. This explanation accounted for the geometrical congruence of many, but not all, rock trails: the rocks that formed congruent trails must have been carried together by the same ice floe.
However, a well-known experiment in the 1970s called the role of ice into question. As part of a long-term survey of Racetrack, two California geologists, Robert Sharp and Dwight Carey, drove steel rebar into the playa’s surface to form a corral around two rocks, with stakes spaced about half a metre apart around the corral’s circumference. (This was, again, before the area became a protected federal wilderness.) Sharp and Carey theorized that the stakes would prevent a large ice floe from carrying rocks out of the corral. Instead, one rock escaped the corral, while the other remained. How could large ice floes do that?
In 2011 we proposed an alternative “ice-pothesis”. An ice floe might buoy a rock frozen inside, and for sufficiently (and plausibly) thick ice floes, the buoyancy would reduce the friction and possibly bring the wind speed required for movement down to observed values (see figure 1, below). An ice raft could also circumvent the Sharp and Carey result since the floes could be small enough to squeeze out of the corral but still large enough to buoy the rocks slightly. Experimenting in his kitchen sink, Ralph froze a small rock in ice and showed that, in principle, ice-rafting could work.
Like so many ideas before it, our ice raft idea was plausible but unproven, and our time-lapse data were ambiguous. Two more years would pass before we would learn whether we were right.
Joining forces
During a visit to Racetrack in autumn 2012, Ralph and I found a brand-new weather station at one end of the playa. There was a phone number attached and, thanks to the vagaries of radio physics (which provided impressively clear mobile phone reception at that one spot in Death Valley and nowhere else), we were able to call it immediately.
A friendly voice greeted us on the other end. The weather station belonged to Jim Norris, an engineer in Santa Barbara, and his cousin Richard Norris, a palaeobiologist based at Scripps Institution of Oceanography in California. They were trying to unravel the mystery, too, and with permission from the national park, they had set up the station as part of their Slithering Stones Research Initiative. They agreed to talk more about their work, and we began an e-mail correspondence.
The Norrises’ approach was to drill holes in test rocks and insert specially engineered GPS receivers (see image above). These receivers would show when the rocks moved and how fast they went, while the weather station would keep tabs on the winds and rain. When Ralph met the Norris group in early November 2013, he teased them that putting GPS receivers in rocks was “probably going to be the most boring experiment in the history of science”. Even so, their approach complemented ours, and we agreed to keep each other informed about any interesting developments. By this time, though, Ralph and I were used to waiting.
But we didn’t have to wait much longer. Arriving at night for a routine visit on 18 December 2013, Jim and Dick found the playa glistening with an extensive layer of ice. When they returned the next morning, a pond covered the southern third of the playa, but below-freezing temperatures kept the rocks locked in place, stuck in ice sheets a few millimetres thick floating on a pond about 5–7 cm deep. At 10 a.m. on the following day, Jim and Dick were at the opposite end of the playa from the rocks, servicing some instruments, when a light breeze began blowing. When they returned to the playa’s southern end in the mid-afternoon, they saw that more than 70 rocks had ploughed fresh trails in the surface. They had just missed it!
Desperately hoping to see the rocks move in person, they camped another night near the playa and awoke on 21 December to watch the sunrise over Racetrack’s broad, icy pond. Melt pools formed and enlarged as the Sun rose, a mid-morning breeze rippled the pond, and abruptly, at 11.37 a.m. the ice broke up, popping and snapping across its whole extent. And then, finally, the rocks began to move.
The ice, although thin as window glass, formed massive floating sheets that shattered as they made contact with the rocks. Most of the rocks remained motionless, acting like miniature ice-breakers as the ice was pushed past them, leaving wakes of ice shards floating in channels of open water downstream of each rock. But other rocks moved, hesitatingly, under the force of the floating ice, ploughing the murky bottom and shoving the mud out of the way, against the underside of the ice. The splintering ice melted quickly, and in less than 15 minutes, the ice panels were too fragile to move the rocks any farther. Within an hour, the floating ice had melted completely.
The subtle motion was hard to capture on film, but Jim and Dick’s GPS trackers showed the rocks had crept along at a few metres per minute, about the same rate as a crawling baby. GPS-tagged rocks separated by 153 m moved together, at exactly the same speeds and times, scribing identical trails (see figure 2, below).
Later that same winter, Ralph joined Jim Norris on a visit to the playa, and the rocks – recovered from their earlier stage fright – put on another show. This time, their movement was captured with Ralph’s kite-borne camera, and Jim filmed a cantaloupe-sized rock as it moved a few metres, at a distance of more than 30 m from shore. This meant that not only had several observers seen the rock movement first-hand, they had also obtained quantitative documentation of the circumstances. We knew how far the rocks moved, how fast and exactly when. We knew how thick the ice was and how strong the wind.
The answers emerge
It turns out that rock motion is rare because it seems to require a very precise set of conditions: a recent storm and a night-time freeze, followed by light breezes and sunshine to mobilize the ice. When these things combine, the vast momentum of a kilometre-wide floating ice panel can shove the rocks around like a bulldozer. These observations suggest that during Sharp and Carey’s rock corral experiment, a thin ice sheet may have shattered past the rebar stakes to push one rock (but not the other) out. Large, but increasingly fragmented, ice sheets could also account for some of the more puzzling results in Messina’s extensive GPS surveys, by producing trails that were often, but not always, parallel.
By mid-February of 2014 the pond had dried up completely and all the rocks went back to their slumber, baking in the Sun on a dry, cracked-mud playa. Rock trails that formed in a few minutes will probably remain for a decade, until a new pond appears. Always a place of wonder, Racetrack Playa is now a place with a bit of scientific understanding, too.
- To learn more about the Slithering Stones Research Initiative, check out these videos from the Scripps Institution of Oceanography:
- Enjoy the rest of the July 2015 issue of Physics World in our digital magazine or via the Physics World app for any iOS or Android smartphone or tablet. Membership of the Institute of Physics required