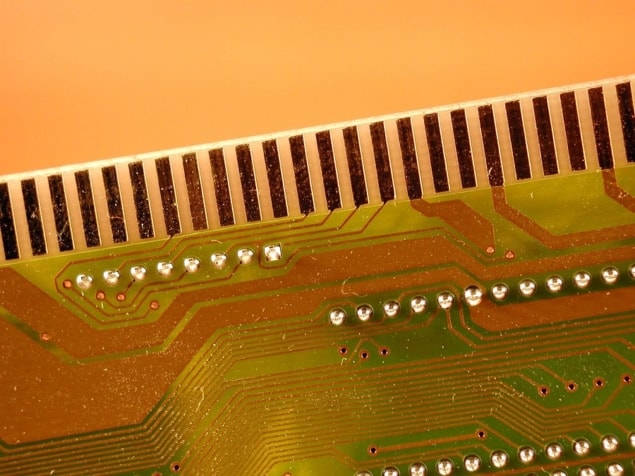
Researchers at TU Delft and University of New South Wales have designed a scalable quantum computing architecture based on widely used complementary metal–oxide–semiconductor (CMOS) manufacturing techniques. The approach encodes information in the spins of individual electrons confined in quantum dots, and could allow the development of large-scale computers incorporating millions of qubits.
Computing milestone
For now, the state of the art in quantum computing is represented by devices with a few dozen qubits: as of November 2017, the 50-qubit, superconductor-based IBM Q is the record holder. Systems of this size are about as complex as can be simulated using a classical computer, so the field has reached an important milestone. But although quantum computers at this scale do have their uses, individual devices will need to harness hundreds, thousands, or even millions of qubits before they really come into their own.
No technology epitomizes the concept of scalability like silicon-based integrated circuits. So reliably and profoundly has this field advanced over the last half-century, that Moore’s Law – essentially just an observation about the regularity of increases in available computing power – has become firmly established in consumer culture. No wonder then that researchers are hoping that the same manufacturing process that has underpinned this growth for so long might do the same for quantum computers.
Small but perfectly formed
Writing in Nature Communications, Menno Veldhorst and colleagues describe how cutting-edge CMOS processes are approaching the point at which silicon microelectronic components can be made small enough to be integrated with quantum-dot spin qubits. The architecture designed by Veldhorst and his team is based on a silicon qubit layer enriched with silicon-28. Above this, and separated by a silica interconnect layer, the classical control and readout circuits would be patterned in isotopically normal silicon. Working at a temperature below 1 K, qubit operations would be controlled by electron spin resonance, coupling by exchange interactions between the confined electrons, and measurement by radiofrequency dispersive readout.
Using minimum feature sizes that are achievable now or anticipated in the near term, the researchers propose a circuit geometry that would result in individual 2D modules of 480 qubits each. Thousands of these modules could be combined, producing a computer containing millions of interacting qubits.
Further reading
Physics World 2015 Breakthroughs of the Year (Dec 2015)
Silicon quantum logic gate is a first (Oct 2015)