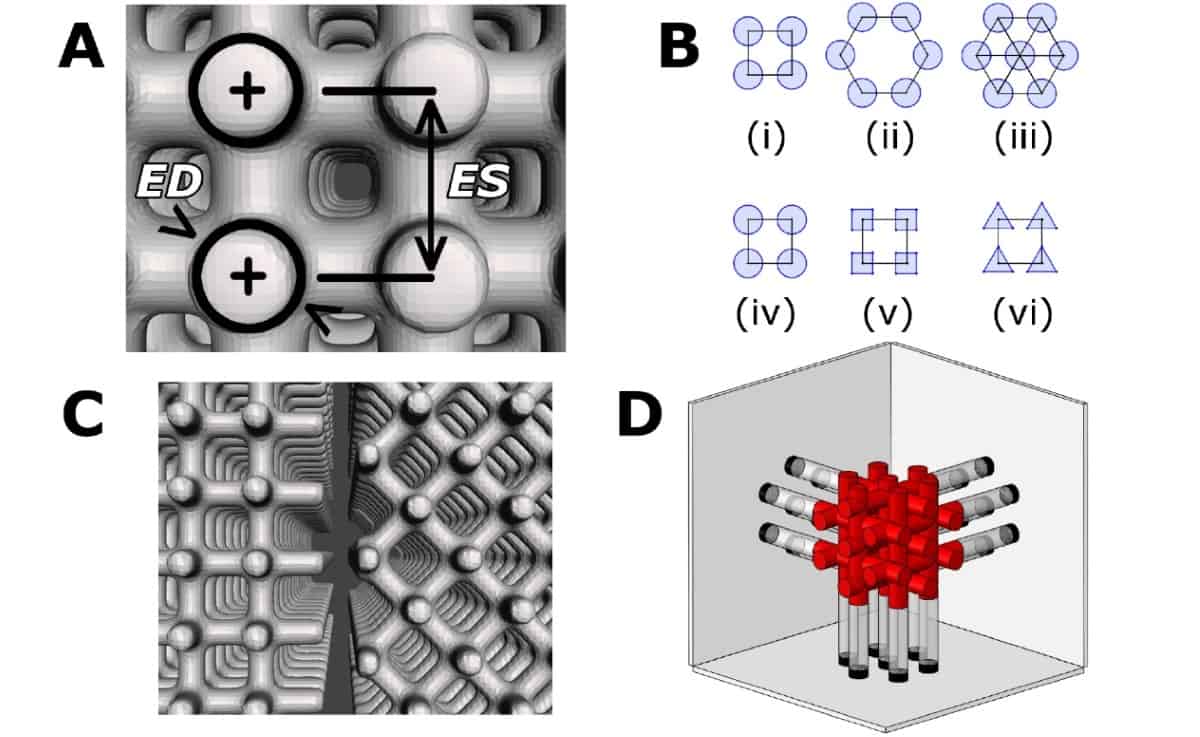
Three-dimensional (3D) printed lattice microstructures that mimic human soft tissues provide a building block towards developing anatomically realistic biomechanical testbed models. Researchers at the University of Washington have developed a method to print a lattice microstructure with the elastic profile of planar fat, the most challenging tissue in the foot to replicate. They have now published details of how varying the lattice properties can affect their stiffness profiles (Bioinspir. Biomim. 10.1088/1748-3190/aae10a).
3D printing of substances for biomechanical human testing could reduce the need for cadavers, which pose inherent difficulties for researchers. Locating a cadaver that has the desired pathologies can be time-consuming and challenging. Healing cannot be simulated. Altering normal specimens to simulate pathology — such as making a cadaveric foot mimic a condition like “flat foot” by releasing tendons and connective tissue — can be labour-intensive and difficult. And surgery on a cadaver may cause irreversible change, preventing an experiment being exactly replicated.
Creating physical model-based biomechanical testbeds by 3D printing, on the other hand, offers the ability to print multiple copies of patient-specific geometries, simulate the effects of healing and integrate sensors. The authors note, however, that materials capable of mimicking the elastic properties of very soft tissues have not yet been developed. For this reason, they are developing lattice microstructures that can increase the compliance of materials currently available for 3D printing.
Principal investigators Eric Rombokas and Patrick Aubin, also from the VA Center for Limb Loss and MoBility (CLiMB), and colleagues, based their method on varying the micro-scale structure of how the material is deposited to vary the macro-scale elastic properties of the object being printed. This enabled them, for example, to create a material with the stiffness profile of the very soft plantar fat.
Tailoring the tissue
The researchers varied five lattice properties: element diameter, spacing, cross-sectional geometry and arrangement, and lattice rotation, to create 14 distinct lattice geometries. Having previously developed a baseline lattice that matched the mechanical properties of planar fat, they then created three to five different samples with incremental changes in one parameter, while the four remaining parameters were held constant.
The team printed the samples using the softest elastomeric material available for their 3D printer. They tested the printed samples using a compression protocol employed in a previous cadaveric study to characterize plantar fat. Each sample was tested for 15 cycles, and the team calculated engineering stress/strain values for all cycles.
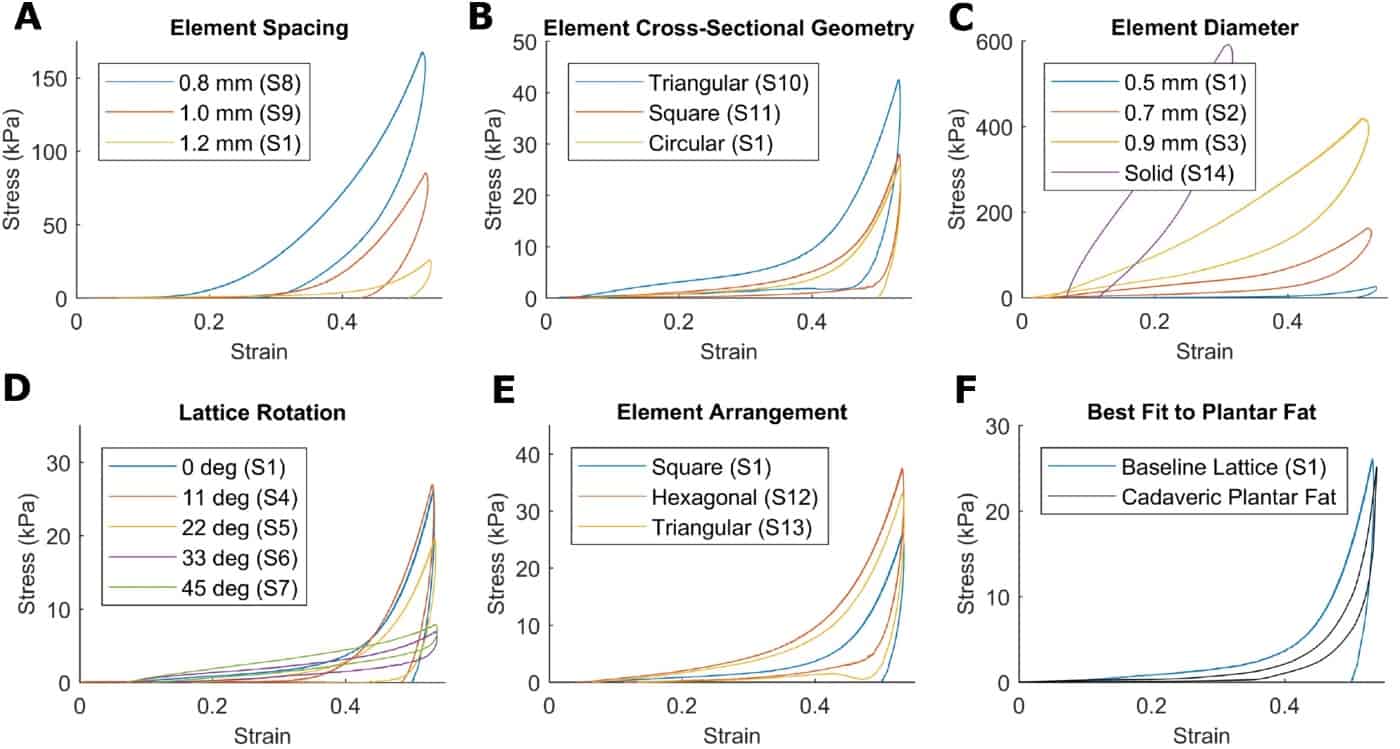
The authors determined that element diameter and element spacing were the most important lattice properties for tuning the stress/strain profile, and that lattice rotation was important in tuning the profile linearity. A square arrangement of cylindrical elements (0.5 mm diameter columns with 1.2 mm spacing) had a stress/strain curve closest to that of cadaveric plantar fat.
This best-fit lattice had Young’s moduli of 7.55, 9.50 and 252 kPa, at 10%, 30% and 50% strain, respectively. By comparison, physiologic plantar fat at the same strain values has Young’s moduli of 1.08, 7.13, and 188 kPa. The authors attribute the discrepancies to different the damping properties of the 3D-printed material and actual fat.
Future plans
The researchers are now experimenting with gyroidal lattice microstructures, which distribute stress and strain very evenly throughout their structure. “We have produced a gyroidal lattice very similar to our plantar fat-mimicking lattice, but dramatically tougher,” Rombokas tells Physics World. “We are using it in some in-progress experiments recreating a full foot from biomedical images, including soft tissue, bones and tendon forces. We’re simulating orthopaedic surgeries and validating the system against cadaveric and computer models. We are also working on creating soft sensors suitable for integration with the latticed tissue models.”
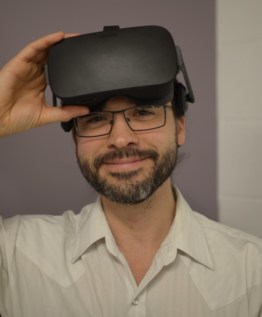
Rombokas explained that lattice microstructures will be valuable for studying targeted subsystems, such as intra-joint pressures in arthritis of the big toe. “Currently, joint-preserving surgeries are diverse and it can be difficult to choose the appropriate procedure,” he explains. “By making identical copies of a particular patient geometry and tissue profile, varying only in the surgical intervention, we will be able to better predict outcomes and inform the surgeons.” And by using thin-film force/pressure transducers in the intra-joint space, it should be possible to compare different surgeries in terms of relief of peak pressure and moving the pressures onto regions of the joint with healthy cartilage.
“Research using lattice microstructures is the next frontier in allowing us to simulate soft tissues,” Rombokas predicts. “The ingredients for a truly transformative robotic biomechanical model are steadily becoming possible. These robots will allow us to answer new kinds of questions. I also think they will have tremendous use outside of fundamental research, potentially as prosthetic limb technologies or robotic agents that can interact seamlessly with human biomechanical systems.”