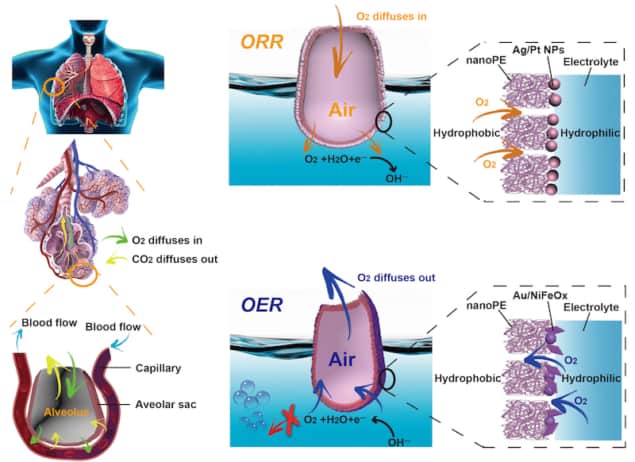
When we breathe, air moves through the small passage-like bronchioles of our lungs until it reaches the alveoli. These tiny sac-like structures contain a micron-thick hydrophobic epithelial membrane on the inside and a hydrophilic one on the outside. This special structure ensures that air does not directly diffuse into the bloodstream, which would create unnecessary (and harmful) bubbles, but allows for efficient two-way gas exchange between the bloodstream and the lungs. A team of researchers at Stanford University in the US has now designed a new catalytic system that works in the same way as our lungs to cycle between water and fuel via the oxygen evolution- and oxygen reduction reactions (OER and ORR). These reactions play a key role in clean energy technologies, such as fuel cells and metal-air batteries, and the new system could help make them more efficient.
“The mammalian breathing process could be mistaken as being simple since it is so natural, but it is actually one of the most sophisticated, nature-designed systems for two-way gas exchange,” explains study lead author Jun Li, who is a graduate student in Yi Cui’s group at the Department of Materials Science and Engineering at Stanford. “The alveoli contain a micron-thick membrane that repels water molecules on the inside while attracting them on the outer surface. It is this unique structure that prevents air bubbles from forming and makes air exchange highly efficient.”
“Our electrocatalytic system structurally mimics the alveolus and carries out two different processes. The first, the OER, is analogous to exhalation, in which water is split to produce oxygen and hydrogen gas (a clean fuel) by oxidizing water molecules in the anode of a battery while reducing them at the cathode. Fast gas transport helps to eliminate the energy of O2 bubble formation and this reduces the overpotential,” says Li.
“The second, the ORR, mimics the inhalation process, in which gas reactant is efficiently delivered to gas-liquid-solid contact lines for enhanced catalytic reaction and reduced gas-diffusion resistance. Here, energy is generated through a reaction that consumes oxygen.”
Pouch-like nanoporous hydrophobic PE membrane
The alveolus in the new system is a pouch-like nanoporous hydrophobic, 12-micron-thick polyethylene (PE) membrane, which is around 30 times thinner than conventional gas-diffusion electrodes. The researchers made this structure by first depositing a layer of catalysts on one side of the PE membrane. These also serve as a charge-transport layer and a hydrophilic coating. They then roll the catalyst-coated PE membrane into a pouch structure with a gas phase inside and a liquid phase outside.
Since the PE membrane is hydrophobic, it prevents water from flooding through, which allows for fast transport of gas molecules and charges – just like in mammalian alveoli. The alveolus-like PE structure creates a barrier that water cannot penetrate but is accessible for two-way gas transport. The added advantage is that it also remains hydrophobic for a substantially longer time than conventional carbon-based diffusing layers.
Record-low overpotential
During the OER, the newly formed O2 molecules quickly diffuse from the catalyst/electrolyte interface towards the gas phase without the additional energy costs of forming bubbles in the electrolyte. Again, like nature’s alveoli. Indeed, Li and colleagues say they were able to achieve a record-low overpotential of 190 mV at 10 mA/cm2 using catalysts made of Au/NiFeOx.

Improving electron transfer in enzymatic fuel cells
During the ORR, O2 gas can transport to the catalyst interface, so overcoming oxygen’s low solubility in water. “The result: around 25 times higher current densities for catalysts made from the Ag/Pt bilayer nanoparticles when compared to conventional flat structures, for example,” says Li. “This breathing mimicking design boasts efficient three-phase catalysis with a minimal catalyst thickness.”
A unique gas exchange system
“It could be easily applied to many other multi-phase catalytic systems, particularly gas evolution reactions and gas harnessing reactions, she tells Physics World. “Gas usually does not dissolve well in an aqueous electrolyte – in a two-phase reaction, for example – which limits mass transport for the reactions taking place therein. Our device is a highly efficient and unique dual direction gas exchange system at liquid-solid-gas three-phase contact lines and promotes mass transport for both gas delivery and release.”
The researchers, reporting their work in Joule 10.1016/j.joule.2018.11.015, say they will now be tuning their catalytic system to fit with different electrochemical reactions. “We also believe that the nanoPE membrane can be replaced with other nanoporous hydrophobic membranes that are stable at higher temperatures,” says Li. “Finally, the membranes themselves can be integrated with other, hydrophilic, nanoporous films (for example, cellulosic material) to allow for fast water adsorption by capillary action for continuous reaction processes and active electrocatalytic reactions at the three-phase interface.”