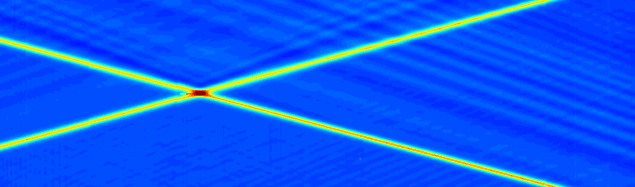
A “gas” make from soliton waves has been created for the first time by physicists in France and Italy. The gas was generated inside a shallow wave tank, where individual solitons could ricochet back and forth. The measured properties of the gas match those predicted by computer simulations and the work could improve our understanding of how waves propagate within a variety of real-world systems.
Solitons are wave packets that maintain their shapes and sizes as they propagate over time – even when colliding with each other head-on. First observed in 1834 as water waves on the surface of a canal, solitons can occur in different media – including optical fibres, where solitons take the form of pulses of light.
A soliton resembles a particle because it maintains its shape and size as it propagates. As a result, an ensemble of solitons can be described as a gas of particles. Soliton gases are thought to play important roles in the dynamics of wave propagation in optical fibres, plasmas and water waves in shallow ocean basins.
Testing times
While soliton gases have been modelled in computer simulations, physicists had not been able to test these calculations in the lab. As a result, researchers do not have a good understanding of how wave-damping effects such as friction and viscosity affect soliton gases.
Now, Nicolas Mordant at the University of Grenoble Alpes and colleagues have created an experimental soliton gas in a shallow 34 m-long wave tank – taking advantage of the fact that water waves can behave as solitons at shallow depths. A piston at one end of the tank generates a steady train of waves, which reflect back and forth across the tank. By fine-tuning the amplitudes and distributions of the waves, the researchers were able to maintain a dense, steady-state soliton gas inside the tank.
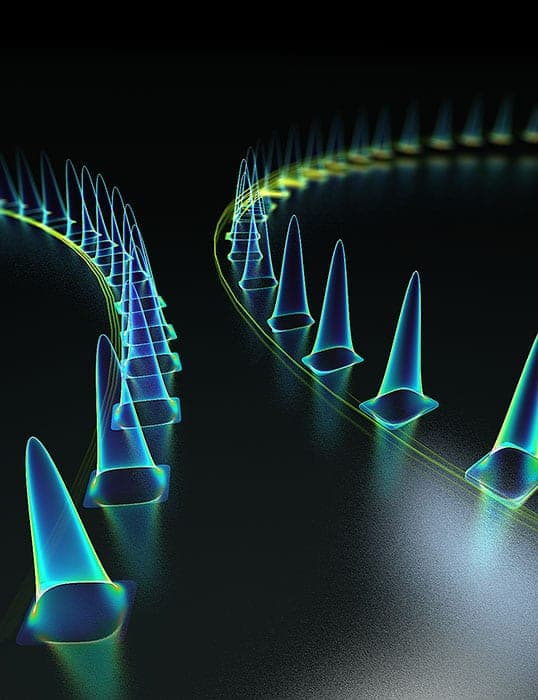
Soliton molecules dance in a kaleidoscope of light
The highly controllable conditions of the setup mean that Mordant and colleagues can make very precise measurements of the entire spectrum of possible excitations within the tank. Across the spectrum, the researchers observed that although the amplitudes of the solitons inevitably decayed over time due to friction, they maintained their profiles, even after colliding with other solitons travelling in the opposite direction. In addition, the measurements of the wave heights in space and time remained extremely consistent with those predicted by previous statistical models of soliton gases.
The researchers say that their experiment is ideal for investigating the special case in which time-varying wave dynamics are entirely determined by their initial conditions – the case of “integrable wave equations”. Such analysis would allow for new insights into the dynamics of systems including the propagation of light along optical fibres, the complex interactions characteristic of plasma waves, and patterns of waves in the ocean.
The research is described in Physical Review Letters.