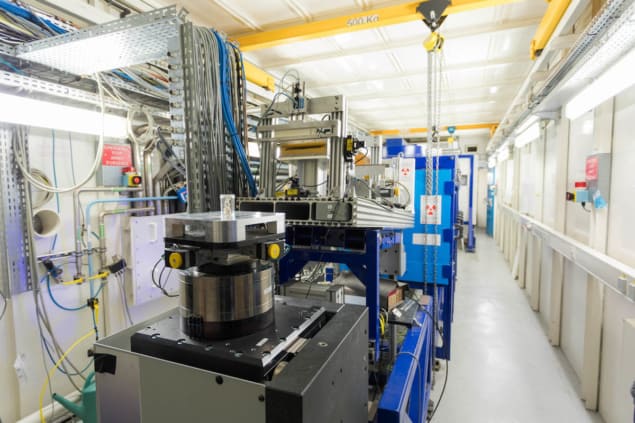
Microbeam radiation therapy (MRT) is an emerging preclinical modality that could one day become a preferred treatment for inoperable brain tumours, due to the reduced impact of microbeams on healthy surrounding tissues. However, the effectiveness of the treatment could be compromised by the effect of brain motion on the precise delivery of radiation dose.
Researchers from Australia and France have conducted a study to assess this possibility using a dosimeter based on a novel single silicon strip detector (SSSD). They determined that the radiobiological effectiveness of MRT dose distribution could indeed be impacted by brain motion (Med. Phys. 10.1002/mp.13899).
The brain is known to exhibit cardio-synchronous pulsating motion, due to expansion and contraction triggered when an arterial pulse travels into the cerebrovascular system. This motion has an amplitude of 100–200 μm, comparable to the microbeam width and spacing, potentially resulting in dose blurring due to microbeam displacement and overlap.
The underlying radiobiological advantage of MRT relies on high peak doses and low valley doses – or a high peak-to-valley dose ratio (PVDR). If microbeams overlap, this can reduce the PVDR and affect the efficacy of the MRT. Building upon prior research, which found that PVDR decreases and full-width at half-maximum (FWHM) of the peaks increases as a function of brain motion amplitude, the researchers conducted the first experimental measurement of microbeam profiles under simulated brain motion.
A team at the Centre for Medical Radiation Physics (CMRP) at the University of Wollongong utilized a real-time dosimetry system that they developed for MRT, based on a SSSD with a spatial resolution of approximately 10 μm. This was placed in a water-equivalent phantom and scanned through the microbeam distribution. The sample positioning stage reproduced brain motion at vertical scan speeds of 5, 10 and 20 mm/s.
The researchers carried out measurements at the ID17 Biomedical Beamline at the European Synchrotron Radiation Facility (ESRF) in France. They employed microbeams with a width of 50 μm and centre-to-centre peak distance of 400 μm, using a tungsten carbide multislit collimator to spatially fractionate the incoming beam.
Mitchell Duncan, a PhD student at CMRP and his principal supervisor Marco Petasecca chose three positions within the dose distribution that corresponded to regions largely, moderately or minimally affected by motion. They reconstructed dose profiles from the SSSD for each scan speed and at each line. They determined that brain motion introduced large disturbances in the dose profile, particularly at low scan speed. At scan speeds of 5 and 10 mm/s, they saw a decrease in total dose delivered, with distorted and broadened distribution.

“When brain motion is applied, the SSSD shows a 150–200% increase in FWHM of profiles and a 50% reduction in PVDR,” the researchers write. “Motion-induced misalignment and distortion of microbeams at treatment delivery will result in a reduced PVDR and increased irradiation of additional healthy tissue, potentially compromising the radiobiological effectiveness of MRT.” They note that at a higher scan speed of 20 mm/s, this effect was drastically reduced, with minimal impact on PVDR and FWHM of the microbeams.

“At the Centre for Medical Radiation Physics, we are mainly dedicated to developing innovative radiation dosimetry sensors and instrumentation for radiotherapy,” says Petasecca. “Microbeam radiation therapy is a particularly challenging radiotherapy modality because it requires dedicated detectors to perform accurate dosimetry of the thin (50 μm width) blades of light spaced by only 400 μm.”
Petasecca explains that his group is studying a new organic semiconducting material for dosimetry. “We are conducting this research because although the SSSD is a very effective and accurate device for MRT dosimetry, its substrate is silicon,” he says. “This is a limitation because the energy deposited in silicon is different from tissue-equivalent materials such as water, particularly in the energy spectrum adopted in MRT.”
“The new organic semiconducting material has the same density as water and has no perturbation of the beam with respect to human soft tissues. We are working to optimize the composition and geometrical structure of this new sensor, to maximize its performance as a dosimeter and bring this game changing technology to the service of the radiotherapy medical industry,” Petasecca tells Physics World.