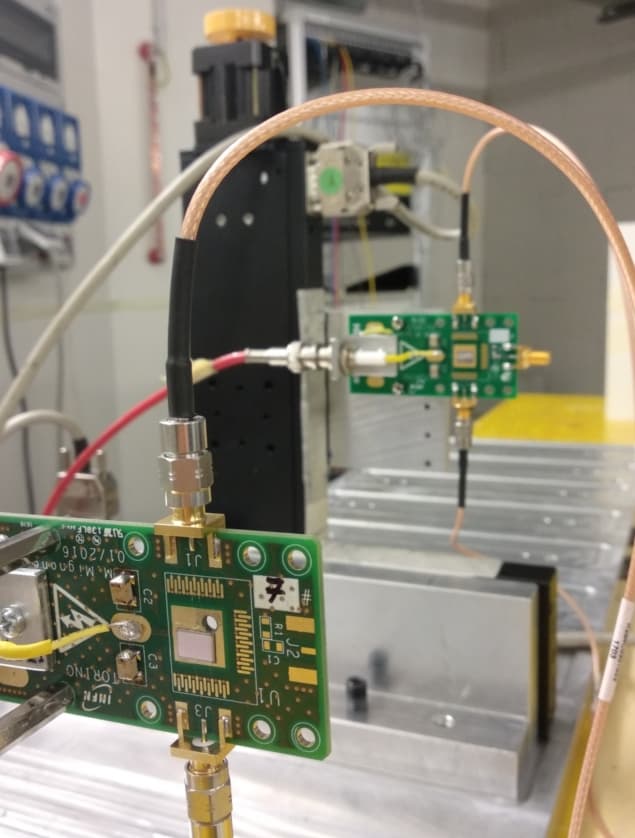
Beam energy is a key parameter in particle therapy, defining the depth inside the patient at which the therapeutic radiation is deposited. Any deviations in energy will change the particle range, which could lead to tumour underdosage or overdose to normal structures, particularly for the increasingly prevalent pencil-beam scanning treatments.
A detector that can rapidly measure particle beam energy could prove invaluable. Such a device could be used for regular quality assurance (QA), to check beam energy during irradiation, or even to implement future adaptive dose delivery schemes employing fast energy modulation. With this in mind, researchers in Italy have developed a prototype detector that measures the time-of-flight (ToF) of protons to determine the beam energy.
To measure the energy, and thus the range, of a clinical proton beam, a detector must match the clinically acceptable range uncertainty, which is typically less than 1 mm at therapeutic proton energies. This corresponds to measurement accuracies ranging from about 0.5 MeV for 230 MeV protons to 1 MeV for 60 MeV protons. To achieve this, the researchers – from the University of Turin and INFN – created a detector from two thin ultrafast silicon detector (UFSD) sensors placed a specific distance apart along the beam direction.
“The main advantages of ultrafast silicon detectors over traditional silicon ones are excellent time resolution, reduced thickness, good signal-to-noise ratio and short signal duration,” explains first author Anna Vignati. “These features allow identification of single protons, even in beams of high intensity and irregular time structure. Thus, the number of protons needed to measure the beam energy with the required accuracy could be collected in a favourable timeframe (a few seconds) for QA checks in a proton therapy facility.”
Vignati and colleagues tested the prototype device on a clinical proton beam at the CNAO facility and reported their findings in Physics in Medicine & Biology. They performed ToF measurements at five clinical beam energies (58.9, 77.6, 103.5, 148.5 and 226.1 MeV, corresponding to water equivalent depths of between 30 and 320 mm). They measured each beam energy with the sensors positioned at four distances, from few centimetres up to roughly 1 m apart.
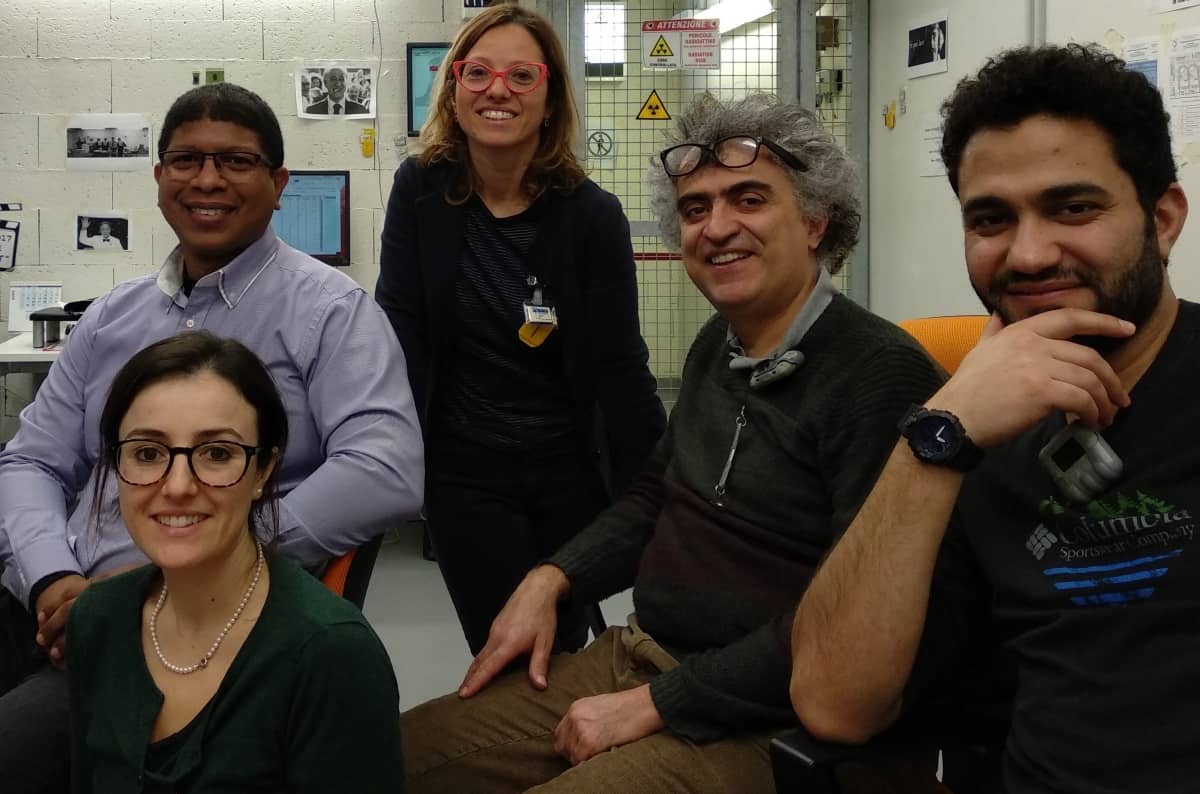
The researchers calculated the ToF from the mean time difference between the coincident signals generated when a single proton crosses both sensors, minus a constant time offset related to signal routing. To determine the beam energy from these ToF measurements, they developed a model that accounts for energy loss in the sensors and in air, benchmarking it against Monte Carlo simulations.
The main error sources for this setup are the uncertainties in the distance between the sensors and the time offset. To minimize these uncertainties, the team developed a calibration method using 16 of the ToF measurements (excluding those at 103.5 MeV, which were used to test the calibration) and assuming the nominal energies at the isocentre as known quantities.
Comparing the measured energies with nominal beam energies revealed that, at the two largest sensor spacings (67 and 97 cm), deviations were less than 0.5 MeV for all five proton energies – compatible with the clinically acceptable measurement accuracy.
The detector also demonstrated short acquisition times. For a test with 97 cm detector spacing and a beam energy of 226.1 MeV, the researchers found that 6 s of irradiation at an intensity of 5×108 protons/s was sufficient to remain below the maximum acceptable ToF error (4 ps for sensors 1 m apart).
From a clinical stance, the most significant parameter is the corresponding range deviation in water. At 67 and 97 cm sensor spacing, the range discrepancies between measured and nominal beam energies were within 0.5 mm at lower energies and within 1 mm for the maximum energy, in compliance with clinical requirements.
The researchers conclude that UFSD silicon sensors can measure the energy of a clinical proton beam in a few seconds, with good accuracy and minimal beam perturbation. They note that their detector could find immediate application for daily QA. “With further improvements, our detector will be even compatible with use during treatment,” Vignati tells Physics World. “Indeed, properly exploiting the extremely reduced thickness of these sensors would minimize the beam perturbation, while reducing the acquisition dead time would allow measurements of similar sensitivity in a few milliseconds.”
To further improve the collection of coincident protons, the researchers have produced a dedicated sensor with appropriate segmentation of the active area. They have also developed a mechanical system that varies the distance between sensors with high accuracy, enabling a self-calibration process that no longer requires knowledge of the beam energy used for calibration. “This ensures that the same precision and sensitivity will be achieved in any clinical facility where the device will be used,” says Vignati.