
A team of researchers from the University of North Florida’s Atomic-LEGO laboratory together with researchers at the University of Illinois are the first to have observed asymmetric ferroelectricity in engineered crystals made from oxide heterostructures. The effect could be used to design nanostructured materials with tailored electronic properties.
Ferroelectricity was discovered a hundred years ago and occurs in some naturally occurring crystals. It is now exploited in a wide range of technology applications, including digital information storage and neuromorphic computing.
Ferroelectric materials have permanent electric dipole moments in the same way that their ferromagnetic cousins have permanent magnetic dipoles. The advantage of ferroelectrics is that their dipole moments can be oriented using electric fields, which are much easier to create than the magnetic fields used to manipulate ferromagnetic materials.
Ferroelectrics usually have two stable states with two equal and opposite electric polarizations. A team of researchers led by University of North Florida’s Maitri Warusawithana has now discovered a completely new phenomenon, however, in which there are two unequal stable polarization states. They found this asymmetric ferroelectricity, as they have dubbed it, in engineered crystals made from oxide heterostructures with three atomically-thin molecular components stacked on top of each other.
Breaking inversion symmetry
The researchers observed the new effect by arranging the stacking order of the molecular layers in a way that breaks inversion symmetry. While the high-temperature crystal structure of any naturally occurring ferroelectric preserves inversion symmetry – the coordinates of the crystal lattice are unchanged when reflected over a symmetry centre – the new engineered crystals break inversion symmetry at all temperatures.
“Breaking inversion symmetry is a necessary condition for electronic polarization,” Warusawithana tells Physics World. “We find that these crystals, by-design, are polarized even at high temperatures. As the temperature is lowered, our electrical measurements reveal that they display an unusual bistable response with two unequal polarization states – an asymmetric response governed by their built-in lack of symmetry at high temperatures.”
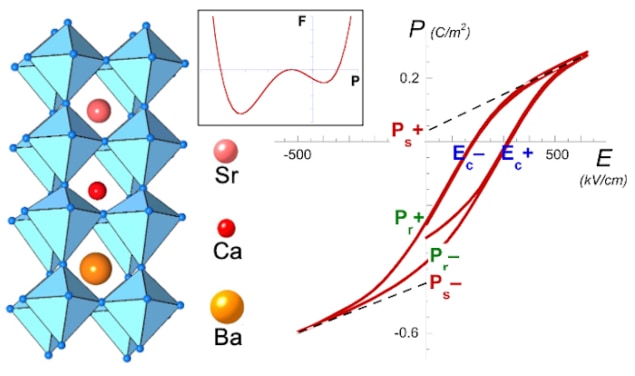
Symmetry is a very important concept in physics and is a fundamental way of expressing its laws since it is related to the conservation of quantities such as energy, momentum and charge. Symmetry breaking is equally as important though and can make a system more complex. An everyday example of symmetry breaking is water, which looks the same in all directions in its liquid state but the same in only six directions when it undergoes a phase transition and becomes a snowflake.
In their experiments, Warusawithana and colleagues introduced strain into a “superlattice” made of stacked dielectric and ferroelectric titanate phases of CaTiO3 (CTO), SrTiO3 (STO), and BaTiO3 (BTO). In their bulk form these materials all have different lattice constants (the physical dimensions of unit cells in a crystal lattice) and the sequence in which they are stacked controls the symmetry of the strain field along the stacking direction. This leads to an asymmetric energy versus electrical polarization relation.
A different class of material
The researchers monitored the strain in their superlattice using X-ray diffraction and found that it causes the different phases in the system to be clamped in-plane to the lattice constant of an underlying substrate. This produces changes to the out-of-plane lattice constant of each constituent phase, which is what creates a broken inversion symmetry in the stacking direction, they explain. Indeed, both the degree and direction of the polarization asymmetry in these artificial crystals can be tuned by the stacking architecture of the molecular components.

Ferroelectricity: 100 years on
“The new asymmetric ferroelectricity that we have observed describes a different class of material, which may have unexpected applications,” they say. “We hope this work will trigger further experimental and theoretical investigations that will not only lead to a quantum understanding of this asymmetric state but also harness the potential of symmetry breaking at the atomic scale to obtain nanostructured materials with tailored electronic properties by design.”
The new discovery is detailed in Physical Review B.