
At temperatures within a few degrees of absolute zero, the ratio of a material’s thermal conductivity to its electrical conductivity should be proportional to its temperature. This principle, known as the Wiedemann–Franz law, was first formulated in 1853, but as our understanding of condensed-matter physics grew, its scope was amended so that it only applies if the same quasiparticles are responsible for carrying both heat and charge. In quantum materials where electrons interact very strongly, it should not hold.
Or so it was thought. Theorists led by Wen Wang of the US Department of Energy’s SLAC National Accelerator Laboratory and Stanford University have now found that the law should continue to be obeyed within one type of quantum material: the copper oxide (cuprate) superconductors. These materials are known as unconventional superconductors, and they conduct electricity without resistance at relatively high temperatures compared to their conventional counterparts. The finding means that physicists will not have to resort to oversimplified and conceptually problematic assumptions involving quasiparticles or Boltzmann equations when predicting how electrons in these so-called strongly correlated materials should behave.
Modelling fermions as electrons that hop between fixed sites
In their study, Wang and colleagues combined a determinant quantum Monte Carlo (DQMC) algorithm with a technique called maximum entropy analytic continuation and applied it to a Hubbard model of a cuprate material. This model represents electrons as fermions that hop between fixed sites on a lattice and interact with each other when they occupy the same lattice site. It is widely employed for simulating and describing systems in which electrons interact with each other rather than behaving as independent entities, and it contrasts with the alternative, Boltzmann framework that defines electrons as distinct quasiparticles.
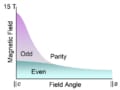
Unconventional superconductor is even odder than expected
The physicists found that if electron transport alone is taken into account, the cuprates’ Lorenz number – their ratio of thermal conductivity to electrical conductivity divided by temperature – approaches the value predicted by the Wiedemann–Franz law. The team suggests that other factors, such as lattice vibrations (or phonons), which are not included in the Hubbard model, could be responsible for discrepancies observed in experiments on strongly correlated materials that make it appear as if the law does not apply. The new results could help physicists interpret these experimental observations and could ultimately lead to a better understanding of how strongly correlated systems might be employed in applications such as data processing and quantum computing.
The team now plans to build on the result by exploring other transport channels such as thermal Hall effects. “This will deepen our understanding of transport theories in strongly correlated materials,” Wang tells Physics World.
The present study is published in Science.