The Mystery of the Missing Antimatter
Helen Quinn and Yossi Nir
2008 Princeton University Press
£17.95/$29.95 hb 292pp
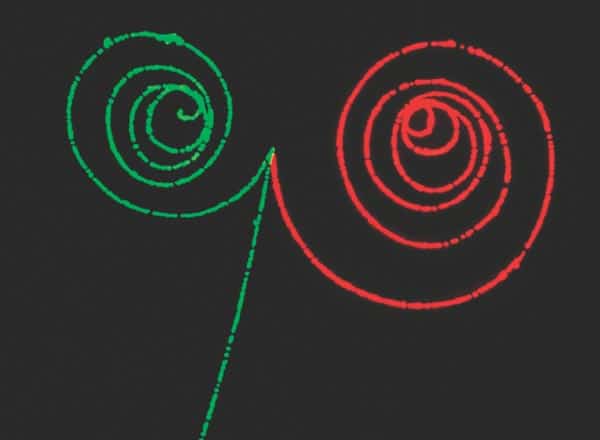
Each charged elementary particle has a counterpart with the opposite charge, which is known as an antiparticle. The antiparticle partner of the negative electron, for example, is the positive positron, which was predicted by Paul Dirac in 1930 and discovered by Carl Anderson in 1932; while for the proton it is the antiproton, which was discovered by Emilio Segrè and Owen Chamberlain in 1955. Just like normal particles, antiparticles can combine, forming atoms of “antimatter”. Dirac’s theory suggested that the laws of physics were exactly the same for matter and antimatter; so given this symmetry, why is our visible universe made of matter with no antimatter? This is the question addressed by Helen Quinn and Yossi Nir in The Mystery of the Missing Antimatter.
A surprising experimental discovery in 1964 suggested a possible answer. While experimenting with K-mesons, which belong to the class of “strange” particles that contain a single strange quark, Jim Cronin and Val Fitch at Princeton University found a small asymmetry between particles and antiparticles. Their experiments revealed that there is an interaction that is not the same for quarks and antiquarks — a phenomenon that now goes by the name of CP violation (where C is charge conjugation and P is parity).
This led the Russian theoretical physicist Andrei Sakharov — who later became famous as a campaigner for human rights — to propose that at the beginning of the universe there were equal numbers of particles and antiparticles, but then, at an early stage in the evolution of the universe, some reaction or decay process that involved CP violation led to the destruction of some of the antiparticles. As the universe evolved further, particles and antiparticles annihilated each other until all the antiparticles were gone and only particles were left. Processes — such as Sakharov’s proposal — that produce an asymmetry between particles and antiparticles are referred to as “baryogenesis”.
Baryogenesis sees elementary particle theory intersecting with cosmology — the history of the universe. This intersection has proved to be a fascinating area of study in recent years as physicists have succeeded in applying known laws to interpret observational evidence about the early universe. However, at times it has also proved necessary to speculate about fundamental laws not yet discovered by experiments here on Earth. The mystery of dark matter, for example, points to some new fundamental physics, and Quinn and Nir discuss this in a quick summary of the standard cosmology of the expanding universe.
The major part of the book, however, is an exposition of the Standard Model of particle physics. The authors provide a systematic introduction to all the particles in the Standard Model, from the strongly interacting quarks to the weakly interacting neutrinos. They particularly emphasize how this model incorporates CP violation and discuss recent experiments at the Stanford Linear Accelerator Center in the US (where Quinn works) and at the KEK laboratory in Japan on particles called B-mesons, which exhibit large CPviolating effects.
Quinn and Nir then discuss how this source of CP violation can be the key to baryogenesis, and this discussion is the most complicated part of the book. This form of baryogenesis depends on a phase transition occurring just after the Big Bang. The authors describe what happens to a phase in which particles have mass and the weak interaction is less active as it expands very rapidly within a hotter phase where all the fundamental particles are massless and the weak interaction is very active. According to the Standard Model, baryogenesis can occur at the surface of this expanding bubble. The result is that there are more quarks than antiquarks inside the bubble, which eventually expands to become the entire universe. This model does not, however, fit with the observational evidence, which has led researchers to conclude that CP violation in the Standard Model is too small to do the job suggested by Sakharov.
In order to solve the mystery, therefore, it is necessary to speculate about particles and interactions not yet discovered. It was originally suggested that the answer might lie with grand unified theories, which predict that at extremely high energies the electromagnetic, weak nuclear and strong nuclear forces are fused into a single unified field. One of the predictions of these theories (which Quinn herself helped develop) was that the proton would decay. Large water-based Cerenkov detectors were built in the US and Japan to search for such decay, but none was observed, and most researchers have abandoned this approach.
The detectors were successful in another respect, however. They were also used to study neutrinos produced by cosmic rays in the atmosphere and neutrinos from the Sun, which led to the discovery that neutrinos have a small mass — contrary to the predictions of the Standard Model (in which neutrinos are massless). The discovery of neutrino masses has inspired new speculation as to the source of the matter/antimatter asymmetry, which goes by the name of leptogenesis.
The basic idea behind leptogenesis is that, besides the three types of neutrinos that have been discovered experimentally, there are three much more massive “singlet” neutrinos, which have no interactions under the Standard Model but instead have a new CP-violating interaction that allows them to decay into more antineutrinos than neutrinos. Under the high-temperature conditions present in the early universe, certain reactions could occur in accordance with the Standard Model that would convert antineutrinos into matter particles, thus converting this neutrino asymmetry into an asymmetry between matter and antimatter.
Future experiments may give hints that this idea is correct; for example, sending neutrino and antineutrino beams to detectors hundreds of kilometres away to try to detect CP violation in the oscillation of neutrinos of one type into another. However, there is no way to directly test these speculations because singlet neutrinos are so massive that they could never be produced in the laboratory.
The Mystery of the Missing Antimatter is extremely well written and easy to read. The style is quite informal, avoiding equations and including occasional personal recollections from Quinn. This means that the authors have needed to simplify sophisticated concepts; however, there is nothing written that is really misleading. The only illustrations in the book are cartoon- like drawings that offer simplistic analogies to physics concepts, such as an elephant sitting on a chair to illustrate forces and energy, and some diagrams or graphs that relate more directly to the physics might have been helpful. Overall, though, the book provides a very stimulating introduction to our current theories of cosmology and particle physics.
And the answer to the mystery? My own belief is that at some point we may have to accept arbitrary initial conditions — in other words, that the universe just started out with an imbalance of matter and antimatter. There may be some questions that have no simple answers. Nevertheless, pursuing questions, even those that may not have an answer, is the only way that science can progress.