A lightweight optic inspired by the structure of crustaceans’ eyes is the key ingredient in a revolutionary new X-ray telescope. Keith Cooper explains how this Mercury-bound instrument could transform high-energy astrophysics
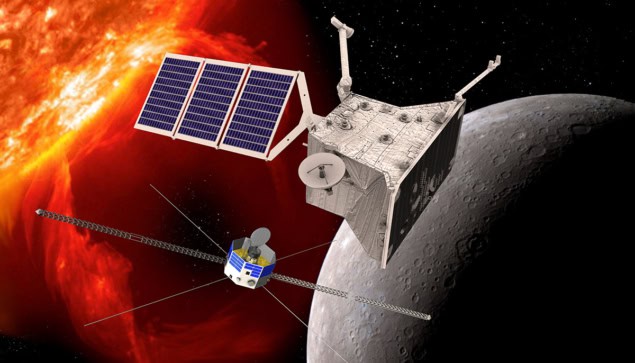
When the BepiColombo spacecraft begins its journey to Mercury in 2018, it will carry a unique payload: the first X-ray telescope bound for orbit around another planet. In the past, such telescopes have been too unwieldy for interplanetary travel thanks to the massive nature of traditional X-ray reflecting optics. With BepiColombo, however, a revolutionary type of optic means that this joint European Space Agency (ESA) and Japan Aerospace Exploration Agency mission could usher in a fundamental change to the way we do X-ray astronomy.
The high energies of X-ray photons mean that they penetrate into and are absorbed by traditional telescope mirrors rather than reflecting off them. To avoid this problem, X-ray telescopes use mirrors aligned along the photons’ direction of travel, which corral these high-energy particles and deal them a glancing blow that sends them towards the telescope’s focus at a shallow angle. Traditionally, these mirrors have consisted of chunky rings of glass or metal shells, but they add substantially to a spacecraft’s mass. Since interplanetary missions have strict mass constraints, X-ray telescopes simply haven’t been able to fly on them.
The Mercury Imaging X-ray Spectrometer (MIXS) aboard BepiColombo gets around this problem with a new design featuring micro-pore optics. Instead of one large glass ring, optics of this type are composed of a series of micro-channel plates: thin glass wafers covered in myriad square holes, or pores, just a few dozen microns across, and coated in reflective iridium. These plates are so light that the entire MIXS instrument has a mass of less than 10 kg – a sharp contrast to the optical system aboard NASA’s Chandra X-ray Observatory, which comes in at a whopping 1000 kg.
Direct comparisons are perhaps a bit unfair, since the two instruments have very different scientific goals. Nevertheless, “The fact that the optics are so lightweight is the only way you can get this type of technology onto an interplanetary spacecraft,” says Adrian Martindale, instrument scientist for the MIXS and a researcher at the University of Leicester, UK. Although an X-ray collimator previously featured on NASA’s MESSENGER mission to
Mercury, Martindale explains that BepiColombo, which is due to enter Mercury’s orbit in 2024, will be the first to carry a bona fide X-ray telescope to another planet. Its job will be to map the composition and elemental abundances of the Mercurian surface, and measure the planet’s auroral zone, where cosmic rays excite elements in the surface to release X-rays.
Lobster eyes consist of many square-shaped cells that reflect light to a focus along shallow grazing angles
The idea for developing X-ray optics along these lines dates back to the 1970s, when Roger Angel, an astronomer at the University of Arizona, US, was inspired by the eyes of lobsters and other crustaceans. While their geometry is not exactly the same as the MIXS system, lobster eyes also consist of many square-shaped cells that reflect light to a focus along shallow grazing angles. However, the lobster-eye technology did not really come of age until the 21st century, when a University of Leicester team led by the late George Fraser began developing it for X-ray observations.
X-ray vision
“George was one of the most brilliant physicists I have ever known,” says Emilie Schyns, micro-channel plates product manager at PHOTONIS France SAS, which manufactures the plates used in the MIXS. Schyns explains that the company’s longstanding relationship with Leicester dates back to the 1990s, when Fraser, who died in 2014, was working in the ultraviolet domain using PHOTONIS’ custom-made micro-channel plates. The micro-pore optics technology on MIXS “evolved out of that working experience”, Schyns says.
The plates manufactured for X-ray astronomy differ from standard micro-channel plate technology in several ways. One is that standard plates are active charge amplifiers with 1000 V running through them, whereas micro-pore optics contain no electronics. Another key difference is the shape of the holes. These are normally round, but to ensure a point-like image at X-ray wavelengths, rather than a diffuse glow caused by photons reflecting in different directions off a curved edge, the holes in MIXS are square.
Currently, PHOTONIS is the world’s only manufacturer of square-pore micro-channel plates. Paul O’Brien, head of Leicester’s Department of Physics and Astronomy, describes the 40-step process of making the plates as “optical cookery”, noting that getting the right curvature for the required focal length involves a proprietary method of heating and moulding the specially chosen glass. The finished plates are then tested using Leicester’s X-ray beam line – the only facility of its kind in the UK, according to O’Brien.
Despite this challenging manufacturing and testing process, micro-pore optics are garnering a lot of attention. Already there are plans for them to appear in the X-ray telescope onboard the French-led Space Variable Objects Monitor (SVOM) mission, which will track down the sources of gamma-ray bursts (GRBs). Although the micro-channel plates don’t have the spatial resolution to pinpoint exactly where on the sky a GRB is, these stellar explosions will be the strongest X-ray emitting objects in that part of the sky, so such accuracy isn’t required.
Meanwhile, a team from NASA’s Goddard Space Flight Center hopes to use the micro-pore optics on a proposed mission that would fly to the International Space Station and hunt for the sources of gravitational waves from a perch on the station’s robotic arm. Similarly, a Chinese proposal called the Einstein Probe hopes to use the optics to find gravitational-wave sources and track down X-ray flares from black holes.
The hunt is on
All three of these missions take advantage of the fact that micro-pore optics can be optimized for wide-field as well as narrow-field observing. This is an important new development for X-ray astronomy, since instruments such as Chandra or ESA’s XMM-Newton satellite only have fields of view of half a degree (about the size of the full Moon in the night sky). The ability to search large swathes of the sky for the sources of gravitational-wave mergers or the afterglows of gamma-ray bursts is “the next big thing in astronomy”, O’Brien says, adding that it is “nice to see technology developed for a planetary mission being used in very different ways”.
The wide field-of-view and limited spatial resolution is not to the detriment of MIXS, since the resolution depends on the number of X-rays detected. However, a different type of optics is required in situations where good angular resolution is important. This is the case for ESA’s upcoming Athena (Advanced Telescope for High-Energy Astrophysics) mission, which is scheduled to launch in 2028 with a next-generation technology called silicon-pore optics on board.
Although the design of silicon-pore optics draws on the same mass-saving principles as the micro-pore optics aboard BepiColombo, O’Brien explains that in other respects, they are very different technologies. Instead of carefully prepared glass plates, the reflecting optics on Athena will be made from silicon wafers, the smoothness of which (down to a few Ångstroms) makes them perfect for reflecting X-rays. Using this existing technology means that “we can leverage the large investments that the silicon wafer industry has already made”, says Giuseppe Vacanti of Cosine Measurement Systems, a Netherlands-based company tasked with developing prototype optics and, potentially, putting them into full-scale production.
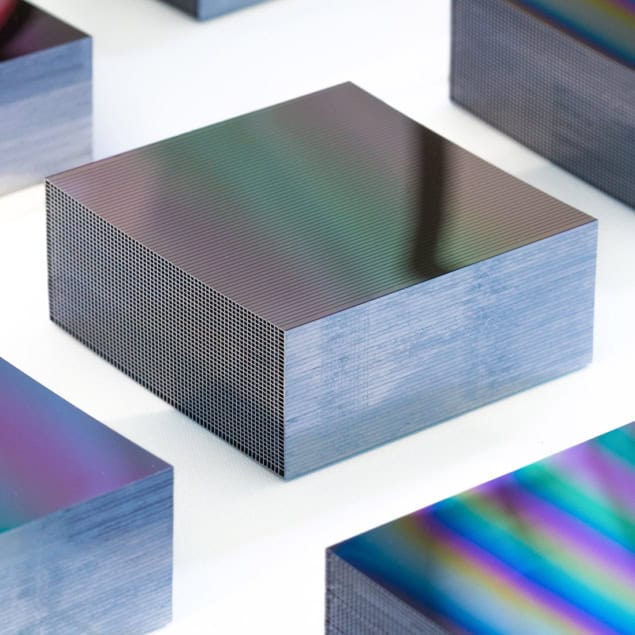
To make the silicon-pore optics, silicon wafers are cut into plates and a series of ribbed channels about 1 mm across are sawn into each one. The sides of the plates opposite the ribs are then coated in highly reflective layers of materials such as iridium and boron carbide, which improve reflective efficiency at higher energies. When these coated plates are stacked together, the ribs act like the holes in micro-channel plates. A silicon mandrel shapes the plates to the required curvature, after which they are stacked together, 35 plates per block, with each block combined with others to form mirror modules. “The difficulty comes in making sure that when you stack them up, you maintain the optical quality and don’t introduce any distortions,” says Vacanti. “It’s more a matter of being careful than the process being complex.”
The prototypes currently have angular resolutions of around eight arcseconds, but Cosine hopes that further refinements to the manufacturing process will reduce this to four or five arcseconds. This would be considerably short of Chandra’s 0.5 arcsecond resolution, but enough to resolve almost all of the X-ray sources that Athena can detect, and with a much larger collecting area. The company aims to demonstrate a finished prototype with four to five arcsecond resolution by 2019; move into mass production the following year; and complete all of the mirror modules by 2025, three years before launch, to allow time for assembly and testing. These are ambitious goals, however, and to meet the deadline Vacanti says that they will have to stack one plate every two minutes – a task that currently takes up to 20 minutes. “The challenge is to carefully place the plates in their stacks fast enough but to not lose any of the precision that we have achieved,” explains Vacanti.
Mission possible
Like the micro-pore optics, silicon-pore optics are receiving interest from elsewhere. NASA is considering a silicon-pore optics-based mission called Arcus, which would use X-rays to study everything from interstellar dust to black holes and galaxy formation. The team at Leicester is also working on proposals for additional missions featuring micro-pore optics. However, their future involvement on missions outside of Europe is currently uncertain. “The Chinese would like us to be involved with their missions, but that requires the UK Space Agency to fund the instrument that we would build,” says O’Brien. “But of course they’re focusing on European missions, so it’s hard to get money for that sort of thing.”
New applications for both types of optic will likely continue to be developed, opening up fresh possibilities for X-ray astronomy. For example, lightweight optics could be used for novel pulsar-based navigation systems. By detecting X-rays from these spinning neutron stars, spacecraft could become self-guiding, eliminating the need to triangulate with Earth-based listening stations. Scientists in other fields may also take advantage of the small, lightweight micro-channel plates to build portable, tabletop devices that were impossible or too expensive before. “Over the next decade or two there’s going to be a spate of instruments using these optics,” enthuses O’Brien. “All that hard work is now paying off for what is becoming a very successful story.”