James McKenzie reflects on the impact of the solid-state laser, a device in its infancy when it became the subject of one of the first articles in Physics World
I am old enough to remember the first issue of Physics World; it was published the year I graduated from Cardiff University in physics and computing. But for the benefit of younger readers out there, one of the most interesting articles in that inaugural edition covered the rise of the solid-state laser. Written by Bridget Marx from the laser-technology supplier Coherent, the article asked if gas lasers should be considered the cumbersome dinosaurs of the laser industry – and whether they might be replaced by “mammals” like the diode laser or diode-pumped solid-state laser. I’d summarize the article’s conclusion as a rather nervous, “Er, no, not yet in the high-power applications, but new applications will become possible.”
Re-reading the article recently, I could see why Marx was so tentative. Three decades ago, it would have been hard to predict the solid-state laser’s impact. That’s because the device itself was in its infancy, and it had only just begun to find its first major application: compact discs. When Sony introduced the CD player in 1982, with a red laser diode at its heart, it cost $730 and required some very sophisticated electronics to make it all work.
But by 1988 costs were falling due to the scalability advantages of semiconductors, both in the laser and the electronics. At the same time, the product’s incredible sound reproduction quality led to huge demand; CD sales overtook vinyl that same year, and cassettes three years later. Soon, the 12 cm optical disc was the biggest money spinner the music industry had ever seen, as the CDs themselves were so cheap to produce.
A grain of sugar
Laser diodes differ from gas lasers in many ways. Made on a wafer scale using semiconductor techniques, they are as small as a grain of sugar and cheap to make in volume. Most importantly, however, they can be directly modulated at high speeds, using cheap electronics. These differences opened up applications that would have been almost impossible to predict in 1988. That was because of other things happening in micro-computing, communications and materials science that may have seemed incidental to laser diodes at the time, but wound up changing everything.
The 1988 article talked about using blue light obtained via frequency doubling (from 840 nm to 420 nm) to increase data-storage densities. Others, however, were working on a direct semiconductor approach. At the time, this looked improbable, but in 1992 the Japanese inventor and later physics Nobel laureate Shuji Nakamura made the first efficient blue light-emitting diode (LED). Four years later he followed it with the first blue laser.
The first digital video disc (DVD) players, using red diode lasers, had appeared in Japan in 1996, and in the US in 1997. Allowing consumers to watch movie – length videos on a small disc, they were a roaring success, with the price plummeting from $600 to less than $50 by 2003. And thanks to the shorter wavelength blue light (405 nm), we were now offered Blu-ray discs containing yet more information. The laser diode had done it again. By 2008, DVD had replaced magnetic video cassette tapes as the preferred method of distributing consumer video content.
The second revolution
There was another major innovation in the late 1980s that was also to drive laser-diode applications, even if it eventually heralded the end for both the CD and DVD. This, of course, was the Internet, and specifically the combination of fibre broadband in the home and mobile data anywhere else. The World Wide Web was created by Tim Berners-Lee at CERN in 1989, and the subsequent explosion in demand for data led to new applications and markets for the solid-state laser/laser diode.
Today, there are so many places where the solid-state laser is used in optical communications that it is hard to count them all. Key examples include narrow-linewidth, high-speed distributed feedback laser diodes, which transmit data over optical fibres, as well as the various optical amplifiers that facilitate the process. The latter include erbium-doped fibre amplifiers (EDFAs), which use 980 nm and/or 1480 nm pump laser diodes to boost multiple optical signals in the fibre, so that they don’t need to be converted back to the electrical domain and then retransmitted (a particularly tricky thing to do in the middle of an ocean).
Invented in 1997 at the University of Southampton by a team led by David Payne, the EFDA became a crucial component in the rapid growth of the Internet, through its ability to transmit and amplify lots of data. Increasing demand for data means that optical fibre is now being deployed closer and closer to our homes. This is only possible thanks to optical transceivers with laser diodes at their heart.
What comes next
Of course, it is easy to evaluate predictions with the benefit of hindsight. But what will the next 30 years bring for the solid-state laser?
I cannot see the laser diode being replaced in 30 years
Many new uses have been touted, including all-optical data centres, digital laser projection displays, holographic displays, LIDAR for autonomous vehicles and many laser-based medical applications. But I am going to be bold and say that I cannot see the laser diode being replaced by anything else in this timescale. Instead, it will continue to be adapted to new applications. That is how fundamental a technology the diode laser is.
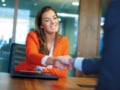
Talent spotting: secrets for a successful job interview in industry
And what about gas lasers? Did they die out like the dinosaurs? In short, no. Although some applications have, as predicted, been taken over by diode-pumped solid-state lasers, gas lasers are still used in many applications and are even sold in greater volume today than they were in 1988. Sometimes even “dinosaurs” live on.