
Thermodynamics was born in the early 19th century, during the age of steam, as railways replaced the horse, and factories mechanized production. Efficiency became the new watchword, and a desire to maximize it in steam-powered machines motivated efforts to understand the flow of heat: the literal meaning of thermodynamics. Quantum technologies, on the other hand, are as futuristic as they come, embodied in the almost caricaturish hi-tech cryogenic coils of the quantum computer.
In her new book Quantum Steampunk: the Physics of Yesterday’s Tomorrow, the University of Maryland physicist Nicole Yunger Halpern shows what emerges when past and future meet. As she sees it, the quantum engines, heat pumps and fridges emerging from the marriage of thermodynamics and quantum mechanics constitute “quantum steampunk”, envisaged here with the Victorian brass-and-mahogany aesthetic found in novels by William Gibson, Neal Stephenson, Philip Pullman and others. The result is a blend of fictional adventure, explanations of quantum science and a glimpse of how working scientists make progress.
It is a fun conceit and, like this book, full of charm. All the same, the topic can sound deceptively modish, because quantum mechanics itself originated in thermodynamics. It was in trying to understand how bodies radiate heat that Max Planck postulated the notion of discretely quantizing the distribution of energy among their atoms in 1900. Planck never intended that to be an expression of physical reality, but Albert Einstein ran with the idea in his 1905 paper on the emission of light and the photoelectric effect. Even here the discourse is couched in thermodynamic terms: Einstein evaluates the entropy of light using Ludwig Boltzmann’s statistical relation.
This connection was never really lost. When Erwin Schrödinger pondered the question “What is life?” in his 1944 book of that title, he expressed it in thermodynamic terms and his answer invoked the probabilistic nature of quantum events and the quantum-mechanical nature of the chemical bond that confers molecular stability.
Both the modern description of thermodynamics – statistical mechanics – and quantum theory confirmed in the eyes of Schrödinger and Heisenberg that nature is fundamentally probabilistic rather than deterministic. And the invention of lasers in the 1950s and 1960s required a consideration of how to populate quantum energy levels in ways that departed from normal thermal distributions, invoking notions of negative temperature.
The general challenge posed by giving thermodynamics a fresh quantum makeover is how phenomena like energy quantization and entanglement – which may strip particles of their independence – change the possibilities for the conversion of heat and work or impact on thermodynamic laws. The second law – the rise in entropy for all irreversible phenomena – is a key focus. British astrophysicist Arthur Eddington captured the common view in 1915 when he said “If your theory is found to be against the Second Law of Thermodynamics I can give you no hope; there is nothing for it but to collapse in deepest humiliation.”
At face value this seems odd, because the usual understanding of the second law makes it too merely probabilistic. It is secure at everyday scales (more properly, in the “thermodynamic limit” of infinitely many particles) simply because its violation by huge numbers of particles has negligible probability. But no fundamental law of mechanics prohibits it: those laws are fully time-reversible. Quantum mechanics too seems reversible, as reflected in the property of the Schrödinger equation called “unitarity”, which basically implies that no information about a quantum system encoded in the equation is lost as the wavefunction evolves. But observation seems to compel non-unitarity and irreversible loss of information. Likewise, it is ultimately loss (erasure) of information that prevents Maxwell’s demon from undermining the second law classically, by collecting and exploiting information about individual particles.
Where quantum meets thermodynamics, then, is really all about information. Classically this may be quantified by the measure of informational entropy proposed by Claude Shannon in 1948. But there is a quantum equivalent, including entanglement, introduced by John von Neumann. In using information to temporarily reverse the second law, Maxwell’s demon reveals how information itself can act as a kind of fuel.
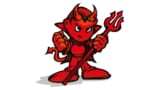
Demons to think with: the rebels you mustn’t ignore
Because quantum mechanics enables us to do things with information that are not possible classically – such as smear it across two or more particles, otherwise known as entanglement – quantum information may fuel new types of machine, such as quantum heat engines. Halpern and her collaborators have devised one such method that uses a phenomenon called many-body localization. Here, quantum effects can seem to stave off the second law for longer than usual by slowing down the process of thermalization that distributes heat from hot to cold.
Like all quantum engines, it involves a cycle analogous to the famous Carnot cycle that launched thermodynamics, cycling between quantum states – in this case, one that thermalizes and one that doesn’t – in ways that generate energy. Quantum batteries, meanwhile, use entanglement to boost energy extraction or charging rates in ways not accessible classically.
As Halpern explains, entanglement here acts as a “resource” that, like coal, can be harnessed to do work – but in non-classical ways. So-called resource theories, which Halpern has helped develop, allow thermodynamics to be recast in a quantum form that does not demand macroscopic averages. These can also furnish experimental predictions about quantum machines, while at the same time offering a new perspective on the second law.
All this is rich soil. But I fear that non-specialists will struggle to follow some of this book. Halpern’s explanations tend to use analogies – sometimes rather contrived or opaque – to describe each step of a mechanism, while failing to convey any sense of the overarching physics involved.
Describing a quantum heat engine as involving photon exchange with heat baths of cold, negative and infinite temperatures, for instance, doesn’t help much without some sense of what roles they are playing or what the overall goal is. Some of the metaphors are over-wrought – appropriately baroque, perhaps, but in the end distracting. Or in some cases mixed or inconsistent: one moment entropy is “liver”, the next it’s a King Charles spaniel.
The narrative is also confused more than it is embellished by framing many of the metaphors using characters and scenes from Halpern’s steampunk novel that may or may not exist in its entirety (it’s not clear which). The scenes are elegantly written, but while we have no sense of the story from which they are drawn, they create a rather arbitrary framework for the book.
Nevertheless, Quantum Steampunk manages to convey some of the excitement and richness in the re-emergence of a quantum take on thermodynamics. Indeed, Halpern shows that, as was the case with quantum information, it is a field that subverts the usual notion of theory preceding and suggesting applications. It’s said of thermodynamics that the theory ended up offering little to the practical questions that inspired it. But for quantum thermodynamics there is often no distinction of “pure” and “applied” at all: new ideas suggest novel devices, but equally, engineering questions are apt to morph into foundational ones. That’s a sign of something profound afoot.
- 2022 Johns Hopkins University Press 288pp £22hb