
Researchers in the US have analysed ash interactions from volcano plumes in a variety of conditions and identified two aggregation regimes – dry and wet – in which particle adhesion is controlled by electrostatic and hydrodynamic forces, respectively. This research – part of a larger investigation into the prediction of eruptive ash dispersal – may act to refine current models of volcanic-plume behaviour. The ability to model the behaviour of the eruptive columns created by explosive volcanoes is much sought after to better understand and predict ash-based hazards.
Volcanic plumes – the kilometres-high columns of hot, upthrust ash created in volcanic eruptions – are among the most dangerous by-products of volcanism, and can have wide-reaching hazardous effects. Columns can collapse under their own weight, forming pyroclastic flows – devastating surges of gas and rock that can reach speeds in excess of 700 km per hour – which can burn and flatten objects in their path. In addition, the ash clouds themselves can pose a serious threat to aircraft, even at hundreds of kilometres away from the eruption site – with ash able to clog up engines and scratch cockpit windows until they become opaque.
Obscure models
Despite being well documented in present day events and past volcanic deposits, the processes of ash aggregation are a major source of uncertainty in current models of eruptive-column behaviour. To model ash behaviour in conditions similar to those found in volcanic plumes, the researchers, based at Georgia Institute of Technology, assembled a controlled atmospheric chamber with dimensions of 15 × 18 × 15 cm. Ash particles were released at the top of the chamber and allowed to collide with a fixed sample – a single layer of ash glued to a glass slide – beneath. These collisions were then observed by means of a high-speed camera.
Collision velocities and kinetic energies of the falling particles before and after impact were estimated, along with the ratio of collisions that resulted in the ash particles adhering to each other. Using two samples of real volcanic ash with different compositions – and one silica-based ash proxy – researchers tested the effect that atmospheric pressures, residence times (how long the ash particles remain in the atmosphere) and humidity had on the ash aggregation.
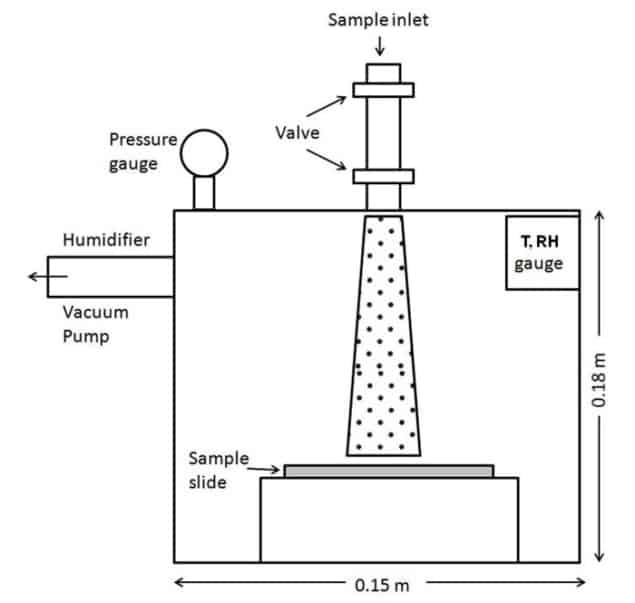
“This study examines the aggregation potential of volcanic ash, or how likely it is for two colliding ash particles to stick together,” explains paper author Jennifer Telling, a geophysicist at Georgia Institute of Technology. “Using the data collected in laboratory experiments, we derive a series of equations to describe the aggregation potential of wet and dry ash particles,” she says. The team observed that while the kinetic energy of the colliding ash particles was the major factor in determining whether or not the particles remained stuck together on impact, humidity also played a significant role. Higher relative humidity (greater than 71%) and long residence times allow the target ash particles to adsorb a larger surrounding film of water from the atmosphere, which acts to hydrodynamically slow down the particles as they impact, thus increasing the likelihood that they stick together. In drier atmospheric regimes, electrostatic forces were seen to be the main force acting to retard the colliding ash particles.
“[This research] is an important contribution to our knowledge of the process of ash aggregation,” says Michael Sheridan, a volcanologist at the University at Buffalo, who was not involved in the study. He comments that total grain-size distribution is a vital parameter in estimating the dispersal of volcanic ash, but that many previous models assume particle sizes remain fixed during transport and deposition. “It is well known that many processes can affect the surface properties of particles between the time of eruption and their arrival at the Earth’s surface,” he adds. “The aggradation model presented here must be seriously considered in future models of dispersion of volcanic ash in an eruptive plume.”
Real-life events
To test the robustness of their modelling, the researchers plan to run a large-scale volcanic simulation. By emulating a specific, real-life eruption event, the output of their numerical models can be compared with the corresponding volcanic deposits in the field to see whether incorporating these new aggregation relationships can improve our predictions.
Explaining that this study represents an important step in the development of plume-dispersal models, Mark Woodhouse of the University of Bristol, who was not involved in this research, told physicsworld.com that “a comparison of predictions of ash-transport models utilizing the products of this research with observations of deposits and ash clouds from volcanic eruptions will be a stringent test of the work”.
Given the dangers and large-scale impacts of eruption columns, the ability to accurately predict the movement and duration of such volcanic-ash plumes could be of great benefit for both hazard mitigation and also to the airline industry. “The models used to predict ash-dispersal patterns are extremely complex,” explains Telling. “We hope that by examining the conditions under which ash can aggregate and its actual potential to stick together, our research can improve the predictive capability of large-scale volcanic simulations,” she says.
The research is to be published in Geophysical Research Letters.