
A new hydrogel binds to spike proteins in the SARS-CoV-2 virus like “molecular Velcro”, preventing it from interacting with potential host cells and inhibiting infection. According to its US-based developers, the hydrogel forms a multi-layer “mask” that abates the action of the virus in a non-specific way – meaning that, unlike vaccines, it would not need to be updated regularly as the virus evolves. The team say the technology could be developed into a cost-effective nose spray that would help fight the spread of airborne infections.
The new hydrogel is made from chains of protein-forming amino acids called peptides. In the latest study, a team led by biomedical engineer Vivek Kumar of the New Jersey Institute of Technology (NJIT) engineered these peptides to self-assemble into a functionalized hydrogel containing nanoscale fibres known as fibrils. It is these fibrils that bind to the protein complexes within viruses – in this case, the spike proteins on SARS-CoV-2, the virus that causes COVID-19.
Targeted vs non-specific approach
The researchers began working on this project in 2020, at the start of the COVID-19 pandemic. By 2021 they had engineered the self-assembling peptides to have a “targeting domain” specific to spikes. In some sense, they say, this mechanism was analogous to the way vaccines produce antibodies by targeting specific proteins on the SARS-CoV-2 virus.
Over the next few years, as new variants emerged, the researchers optimized their peptides further. Then, while performing a control experiment in live-virus assays – one that involved the hydrogel’s self-assembling domain on its own, without the targeting domain – they observed that some peptides could interact with the charged viral protein coat or viral proteins in a non-specific way. “Interestingly, we also found a synergy (improved viral inhibition) when testing combinations of the just-self-assembling domain and the self-assembling domain plus targeting domain,” Kumar says.
Further investigation showed that the self-assembled fibrils act like “molecular Velcro”, forming a stable fibrous mesh on the virus that is also highly resilient to viral mutations, Kumar adds.
“The ability to design and optimize these assemblies and target novel receptors is truly exciting,” says team member Petr Kral of the University of Illinois in Chicago (UIC), “along with the ability to tune densities in functionalized peptides to enhance targeting.”
Simulations and safety tests in laboratory animals
The team tested the fibrils on several SARS-CoV-2 variants – first with computer simulations at UIC, and then in the laboratory on mice and rats via injections and nasal sprays. They found that the treatment inhibited the Alpha and Omicrons variants of SARS-CoV-2 in vitro, while the animals exhibited no adverse effects.
While the hydrogel has not yet been tested in humans, Kumar says the early results are promising. “We think this platform could be expanded to a number of other disease-causing viruses, and could potentially rapidly, and cost-effectively, address the dearth of specific drugs/devices on the market for emerging epidemics/pandemics,” he says. He adds that the hydrogel could be “useful as a therapeutic in early stages of a disease or as a prophylactic – a gel sprayed into the nose to prevent the virus from infecting the host more seriously”.
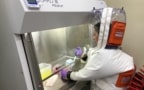
Cellular nanosponges could neutralize SARS-CoV-2
The researchers are now seeking to understand how the fibrils interact with the spike proteins on SARS-CoV-2. In particular, they would like to know whether the infection-inhibiting mechanism is biomechanical in nature. The answer could have important implications for the platform’s versatility. “Drug-resistant pathogens mutate around biochemical modulators, but are they less likely to mutate around a mechanical spear?” Kumar asks rhetorically. “By understanding this fundamental interaction, we want to figure out how to use it against different diseases.”
The team, which also includes scientists from Georgia Tech, the Baylor School of Medicine and Rutgers University, hopes to find partners interested in developing the technology further. “We would like to extend it to other viruses, which we have shown is possible in computational simulations,” Kumar tells Physics World. “We are also exploring expanding the platform to a number of hard-to-treat bacterial and fungal pathogens that we have seen excellent efficacy against.”
The study is detailed in Nature Communications.