China is a big country with a booming economy, so it is no surprise that it is investing heavily in big science facilities. Ling Xin takes us on a tour of four facilities that are in the building stages
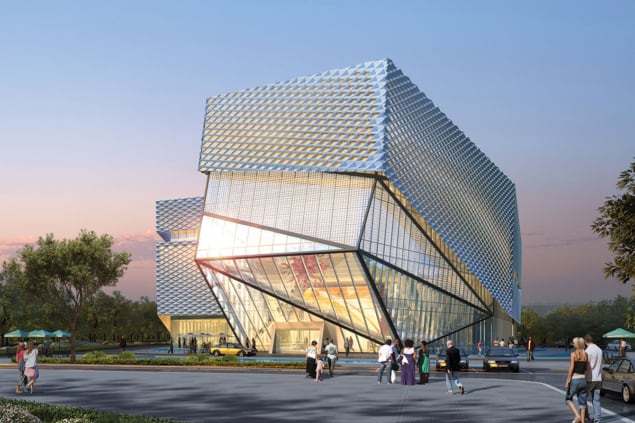
Shanghai will soon be home to one of the world’s most advanced X-ray lasers. Civil engineering of the Shanghai HIgh-repetitioN-rate XFEL and Extreme light facility (SHINE) is on schedule, according to deputy project manager Liu Zhi from Shanghai Tech University, who reports that excavation of all five shafts and part of the 3.1 km-long underground accelerator tunnel have been completed.
Like synchrotron radiation facilities, X-ray free-electron lasers (XFELs) accelerate electron bunches to very high energies. The electrons in an XFEL are sent through a magnetic undulator, which cause them to emit a bright pulses of X-ray photons that are coherent – much like the light from a laser. These X-rays can then be used for wide variety of research, including physics, chemistry, medicine, life sciences, and materials science. Compared with synchrotron radiation facilities, XFELs can generate X-ray pulses a thousand times shorter by time duration and with a billion times higher peak brilliance.
SHINE is capable of taking one million shots every second, making it a “super camera” that can take not only high resolution images of atoms, but also videos to show their dynamic behaviour during physical and chemical processes.
SHINE will comprise a superconducting linear accelerator, three undulator lines, three photon beamlines and 10 experimental stations for the first phase of the project. In the linear accelerator, electrons will receive several “kicks” and be accelerated to nearly the speed of light with up to 8 GeV energy. Then the electrons will be split into the three undulator lines, where X-ray photons at different energies between 0.4 and 25 keV will be created. The photon beams will be fed into experimental stations located in two underground experiment halls.
According to Liu, in one experimental station the X-ray photons will be used together with a 100 PW laser – which will be the world’s highest peak power laser – to study the virtual particles that appear and disappear in the vacuum as described by quantum electrodynamics. In another station, scientists will use the coherent diffraction imaging to determine the structures of non-crystalline materials such as biomolecules, viruses, liquids and plastics.
The major technical challenges in building SHINE include designing and building the superconducting accelerator, the superconducting undulators, and the ultrasmooth mirrors needed for photon transportation and distribution. “Like all the most advanced machines, we are working on cutting-edge technologies that have never been done before,” Liu says.
As the largest research infrastructure China has ever approved, SHINE will cost a total of 9.5 billion yuan ($1.5 billion). Construction is a joint effort by ShanghaiTech University and two research institutes under the Chinese Academy of Sciences: the Shanghai Institute of Applied Physics and the Shanghai Institute of Optics and Fine Mechanics.
SHINE is a key part of the photon-science complex now taking shape in Shanghai’s Zhangjiang Hi-Tech Park, along with the Shanghai Soft X-ray Free Electron Laser Facility, the Shanghai Superintense Ultrafast Laser Facility, and the existing Shanghai Synchrotron Radiation Facility, all located in the same area. “Shanghai will soon join Hamburg in Germany and Stanford in the US to become another world-class photon-science centre,” says Liu.
New spin on geosciences
When you think of big science facilities, particle colliders or nuclear reactors spring to mind – not what will soon become the world’s most powerful centrifuge for scientific research. Located in Hangzhou, in eastern China’s Zhejiang Province, the Centrifugal Hypergravity and Interdisciplinary Experiment Facility (CHIEF) is currently under construction.
CHIEF will create accelerations hundreds or thousands of times stronger than Earth’s surface gravity (1g, roughly 9.8 m/s2). Facilities like CHIEF can reduce space–time scales and study gravity-related phenomena over long distances or time durations in a lab to serve a wide spectrum of engineering purposes, says Chen Yunmin from Zhejiang University’s Institute of Geotechnical Engineering, who is principal investigator of the project. For instance, to examine the stability of a 300 m-tall dam, scientists can make a 3 m-tall model, spin it at 100g, and precisely reproduce the same stress level in the model dam as in the prototype. Such machines also allow researchers to look into the potentially dangerous resonance between the ground and the track of high-speed trains, or the transport of pollutants over tens of thousands of years – all in the laboratory.
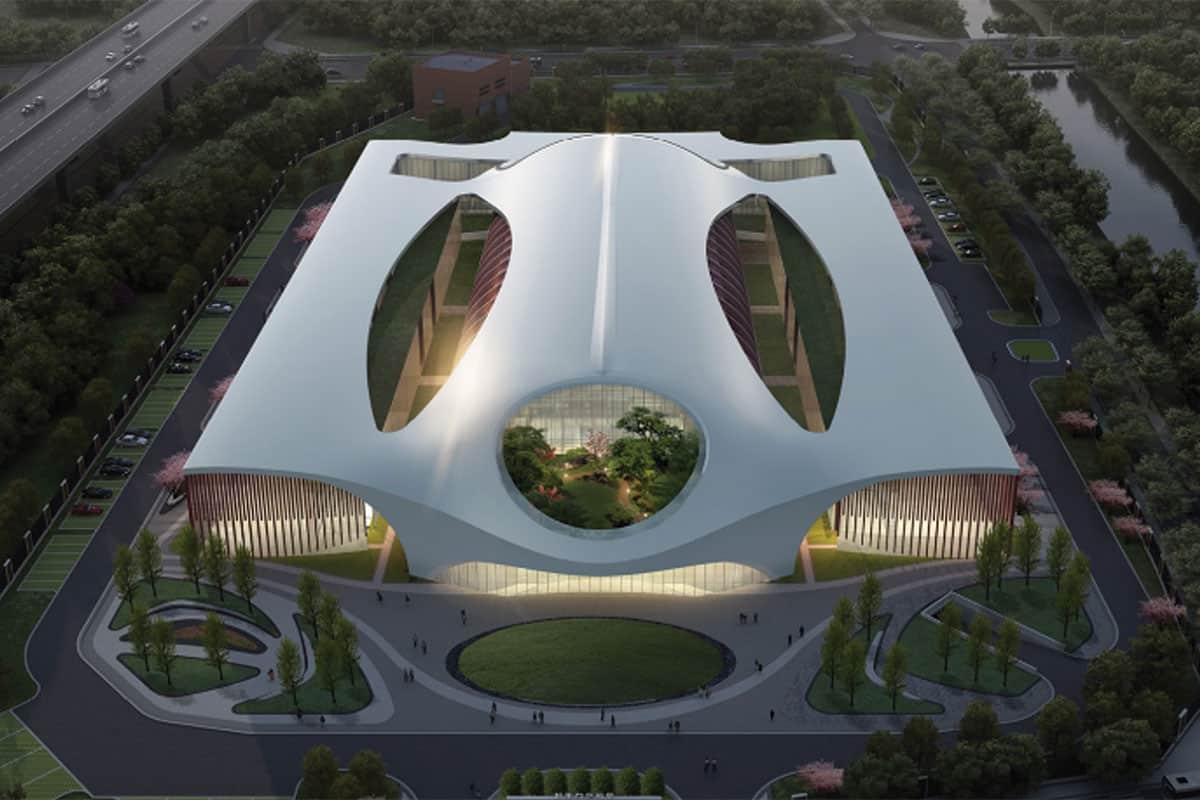
CHIEF will comprise a duo of giant centrifuges. The large-capacity centrifuge will have a pair of 8.5 m-long arms to spin models as heavy as 32 tonnes. With a total capacity of 1900 g/tonne, it aims to mimic geological disasters such as dam collapses, landslides and earthquakes, as well as natural resources exploitation from deep underground or the deep sea. The high-speed centrifuge has 4.2 m-long arms with maximum acceleration of 1500 g. It can mimic geological processes, help scientists better understand the separation of earth’s interior materials, and search for high-performance alloys.
The current world-leading hypergravity facility was developed by the US Army Corps of Engineers with capacities around 1200 g/tonne, Chen says. For CHIEF to achieve a significantly higher load and acceleration, one major challenge is to maintain the machines’ balance and reduce low-frequency vibration during the spin. His team is testing a new hydraulic bearing which will be key to the stable connection between the structure and the centrifuge spindle.
With a total investment of two billion yuan ($308 million), CHIEF will be complete by late 2024 and open to domestic and overseas users.
Chen is already a keen user of the 400 g/tonne ZJU‑400 hypergravity facility at Zhejiang University. In a recent study of soil liquefaction during earthquakes, 40 cm-thick soil models were spun at 50g and ground motion was simulated using an inflight shaker. This allowed the researchers to study liquefaction in 20 m-deep soil. Their results were later verified by the US Geological Survey’s global strong earthquake database, which is a survey of hundreds of earthquake sites around the world in the past 50 years.
“It showed that the hypergravity physical modelling approach is effective and works really well,” Chen says. Centrifuge studies are complementary to computer simulation, he says, and sometimes crucial when our understanding of the system is not sufficient to create a computer model. Compared with ZJU-400, CHIEF will allow payload dimensions nearly five times as large.
Pushing on the nuclear frontier
Delivering the highest-intensity heavy-ion beam in the world is the goal of the High Intensity heavy-ion Accelerator Facility (HIAF), which is under construction in Huizhou in southern China’s Guangdong Province. Earth and rock excavations for the 2.6 billion yuan ($400 m) facility have been completed and HIAF is expected to open in December 2025.
By accelerating heavy ions to very high energies and beam intensities, HIAF aims to push the frontiers of nuclear physics and nuclear astrophysics. For instance, it will explore unknown regions of the chart of nuclides, seeking to synthesize new isotopes and superheavy elements – thereby extending the periodic table.
HIAF will produce and study a variety of extremely neutron-rich and neutron-deficient exotic nuclei to test the boundary of nuclear structures. The facility will also recreate nuclear reactions in stars to study how elements heavier than iron are produced in the universe. This will help explain why some elements are more abundant than others, explains Zhao Hongwei from the Institute of Modern Physics, Chinese Academy of Sciences in Lanzhou, who is deputy manager of the project.
For HIAF to create intense heavy-ion beams, different kinds of atoms – from carbon and oxygen to uranium – will first be stripped of some or all of their electrons and accelerated 100 m in a superconducting linear accelerator. Next, they will enter a 570 m-circumference booster ring and be further accelerated to nearly 1 GeV. The beams will then be transported to either a spectrometer ring or used to bombard fixed targets to generate secondary particle beams.
HIAF is designed to achieve a maximum pulsed heavy-ion-beam intensity of 1 × 1012 ppp (particles per pulse), which will be the world’s highest according to Zhao. It is expected to have a higher intensity than the Facility for Antiproton and Ion Research being built in Germany and the Nuclotron-based Ion Collider Facility soon to be operational in Russia.
To create a design capable of this performance, the HIAF team has overcome major technical challenges related to the superconducting ion source, the superconducting linac, the two-plane painting injection into the booster ring, and the thin-wall vacuum chamber, among others.
HIAF will be used by Chinese and international researchers from a range of disciplines, including nuclear physics, atomic physics, materials science, biology and medicine.
If funding allows in the future, HIAF will add a superconducting ring in parallel with the current booster ring to improve beam energy and intensity, says Zhao. Another major science facility is also being at the same site in Huizhou. It is called the China Initiative Accelerator Driven System (CiADS) and will study the safe disposal of nuclear waste and share research infrastructure and interests with HIAF.
Weighing tiny particles
The civil engineering phase of the Jiangmen Underground Neutrino Observatory (JUNO) is now winding down in southern China’s Guangdong province. “We are ready for the most exciting onsite installation phase,” says Cao Jun from the Institute of High Energy Physics, Chinese Academy of Sciences who is a deputy spokesperson for the project. Starting in mid-2023, JUNO will detect neutrinos from nearby nuclear power plants to determine the neutrino mass ordering and measure neutrino oscillation parameters – among other research goals.
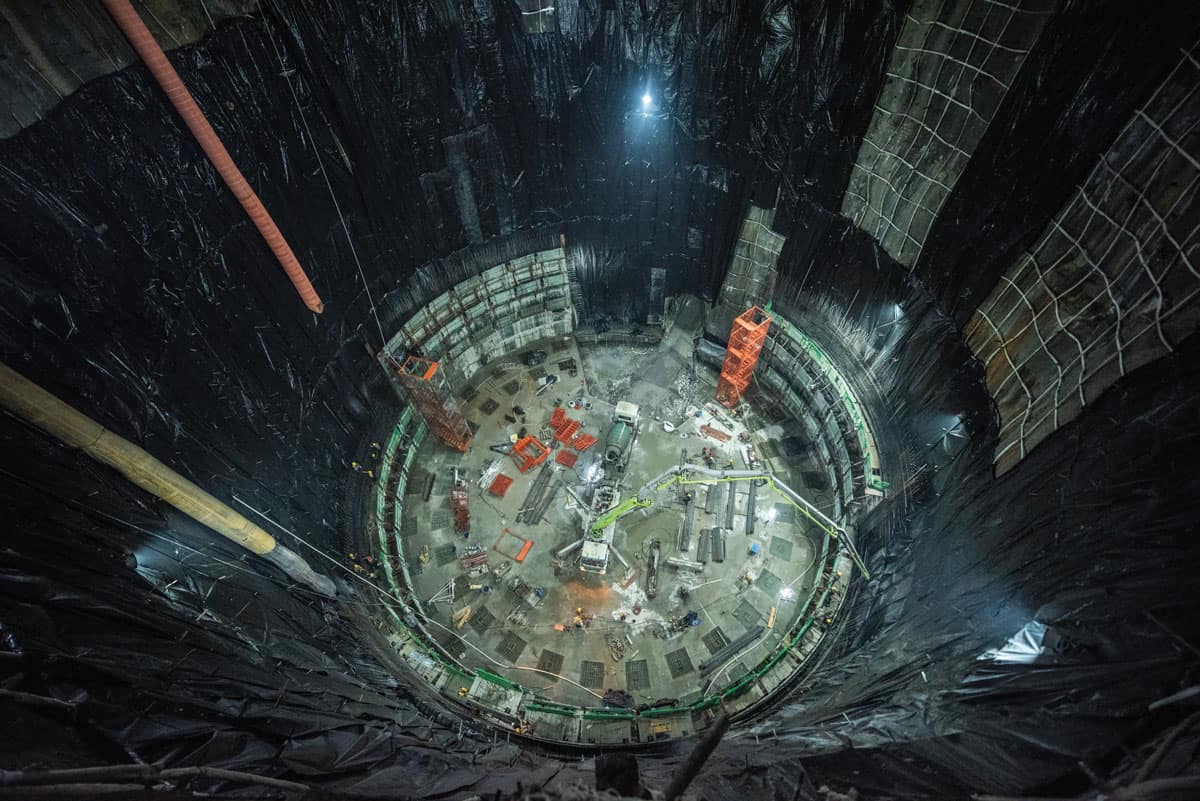
Neutrinos are among the lightest and most abundant elementary particles in the universe. They come in three types, or flavours: electron neutrinos; muon neutrinos; and tau neutrinos. Furthermore, neutrinos oscillate from one flavour to another as they travel near the speed of light, rarely interacting with matter.
This oscillation is a result of each flavour being a combination of three neutrino mass states. Scientists do not know the absolute masses of neutrinos, but studies have shown that two of the mass states are similar. Finding out if the third state is heavier or lighter will help physicists estimate the total mass of neutrinos in the universe and gain a better understanding of their role in cosmic evolution. It could also help in the search for neutrinoless double beta decay, which if observed could provide important clues as to why is universe is dominated by matter instead of antimatter.
To determine the neutrino mass ordering, JUNO will precisely measure the energy spectrum of electron antineutrinos released by Yangjiang and Taishan nuclear power plants, both 53 km away. This will be done using a 35 m radius spherical detector, containing 20,000 tonnes of liquid scintillator. When an electron antineutrino occasionally bumps into a proton in the liquid, it will trigger a reaction that results in two flashes of light. The light signals will be detected by some of the 43,000 photomultiplier tubes that will be observing the scintillator.
“The two nuclear power plants combined can give off 5000 billion billion neutrinos every second, but the chance for them to bump into anything is so low that only about 60 detections will happen in JUNO’s detector every day,” Cao says. Therefore, it will take JUNO about six years to collect enough data to answer the neutrino mass ordering question.
With a total investment of 2.2 billion yuan ($340 m), JUNO is an international collaboration of more than 600 scientists from 17 countries including China, France, Germany, Italy, Russia, Thailand, and the US. It is one of three next-generation neutrino experiments along with the Hyper-Kamiokande in Japan and the Deep Underground Neutrino Experiment in the US. It is also the second neutrino experiment in China, after the Daya Bay Reactor Neutrino Experiment which successfully measured a key neutrino oscillation parameter called θ13 in 2012.
The construction of JUNO started in early 2015, but the project suffered from serious flooding, which delayed the construction for three years. Now the plan is to complete the installation of detectors by the end of 2022, followed by six months of the production and filling of the liquid scintillator. Once operational in the summer of 2023, JUNO is expected to run for about 30 years, Cao says.