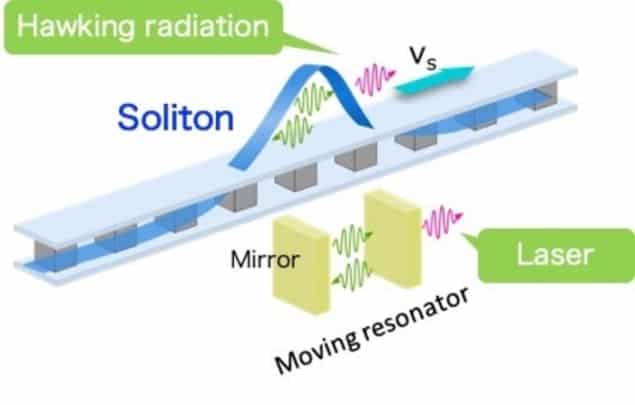
An electromagnetic analogue for a black hole laser – a system that could theoretically amplify Hawking radiation from the event horizon of a black hole and make it observable – has been proposed by Haruna Katayama of Hiroshima University in Japan. The idea follows on from demonstrations of analogues using Bose-Einstein condensates and has the potential to provide new insights into the relationship between quantum mechanics and gravity. If built, the device could even advance technologies such as quantum computing.
Hawking radiation is one of the few hypothetically observable predictions arising when the two great pillars of modern theoretical physics – general relativity and quantum mechanics – bump into each other. At the event horizon of a black hole, quantum mechanics predicts the creation of photon pairs. One of the photons, which has negative energy, disappears into the black hole. The other, which has positive energy, escapes into outer space. This effect would cause black holes to emit radiation, giving them a measurable temperature – which would be theoretically revolutionary as it would suggest they had internal degrees of freedom. Unfortunately, the temperatures of all known black holes would be lower than that of the cosmic microwave background. The radiation emitted would be masked by the radiation absorbed and be unobservable.
In 1981, however, William Unruh, of the University of British Columbia in Canada showed that several physical systems are mathematically identical to that which produces Hawking radiation and therefore the effect could be studied in the lab. Among these analogues are water waves, fibre-optic systems and Bose-Einstein condensates.
Disputed claims
“[These analogues are] not going to get to the heart of any question having to do with quantum gravity because that’s going beyond the regime one is exploring here,” explains theoretical physicist Miles Blencowe of Dartmouth College in the US; “but there are still important questions with Hawking’s calculation. In a way you can think of these analogues as like quantum simulators.” Groups working with different “analogue gravity” systems have competed to produce the first evidence of various predictions of Hawking, and claims by one group have often been disputed by others.
In the new work, Katayama proposes that one of the most eye-catching predictions of Hawking’s theory, made in 1999 by Steven Corley of the University of Alberta in Canada and Ted Jacobsen of the University of Maryland in the US, could be tested in a superconducting electric circuit. The duo outlined the operation of a black hole laser that requires a black hole to have a “white hole” inside it. The inner horizon of this white hole reflects negative energy photons back towards the black hole horizon, where, unable to escape, they are reflected back. The energy of the photons grows ever more negative as it bounces between the horizons, causing the energy of the photons emitted into outer space to become ever more positive.
“It’s very unlikely that one of these could be realized in nature, but it is possible to generate these in analogues,” says Blencowe. Indeed, the first such black hole analogue was produced in 2016 in a Bose-Einstein condensate.
Entangled radiation
In this latest work, Katayama proposes using the Josephson effect, which allows a superconducting current to become quantized, to create a non-dispersive wavepacket called a soliton in a metamaterial resonator. The soliton itself behaves as a resonant cavity, with radiation in the soliton becoming quantum-mechanically entangled with radiation emitted from the soliton. This emitted radiation is the analogue of Hawking radiation.

Entangled Hawking radiation spotted in analogue black hole
“Unfortunately, at this stage we have not been able to make proposals that surpass other [analogue] systems with this system,” says Katayama. “However, the dynamic Casimir effect, which is the dynamic fluctuation of the vacuum, has been revealed based on the proposed superconducting quantum device, and the photon detection technology developed in this system is a great advantage that cannot be imitated by other systems. In addition, this system, which is based on nanotechnology, has good controllability. Therefore, by controlling the circuit parameters, it is possible to bring the black hole from the classical domain to the quantum domain, so it may allow us to study the quantum pair creation of black holes and white holes from a vacuum.”
Blencowe agrees the system’s sensitivity could aid the search for Hawking radiation: “Systems very close to this have been realized: they’re very important as very sensitive detectors of microwave photons and they’re very important in superconducting quantum bits,” he says; “If the proposals are realized it would be a very clean demonstration of the Hawking effect – the signal is relatively large and you wouldn’t have to worry about the noise so much.” Moreover, he sees significant potential for technology transfer: “Quantum computing is all about generating entanglement as a resource, so entangled microwave photons generated through these kinds of systems could be very useful,” he suggests.
The research is described in Scientific Reports.