Deji Akinwande is an applied physicist and electrical engineer who develops flexible electronic devices based on 2D materials. He spoke to Margaret Harris about the challenges of working in this interdisciplinary field
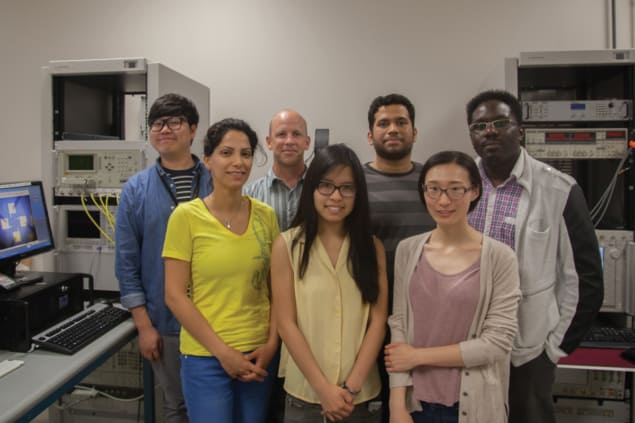
The most famous 2D material is graphene, but the focus of your research has shifted to other substances. Why is that?
We are still working on graphene, it’s just that we have expanded to investigate other 2D materials as well. The value of these other 2D materials and the motivation for investigating them is that they have properties that graphene does not have.
Graphene is unique in its material properties, but other 2D materials are also unique from an electronic, optical or magnetic point of view, so they are very worthy of consideration. It’s like if you have a sports car. It has value if you want to speed down a fast road. But if you want to carry cargo during transportation, you need a truck. These other 2D materials complement what graphene has to offer in a unique way.
A few years ago you used one of those other 2D materials, molybdenum disulphide (MoS2), to make a flexible transistor. What did that project involve?
That was a very multidisciplinary project, which was challenging for us at that time. I’m an electrical engineer and an applied physicist, but that project also involved quite a bit of materials science in terms of materials synthesis. We had to do things from the bottom up, starting with growing the materials ourselves, then putting it on a flexible substrate like plastic kapton tape and creating devices on this non-electronic substance. And then, after fabricating the devices, we had to characterize them and do measurements to ensure they were of good quality. So it was a very complex project, spanning materials science, materials chemistry, applied physics and electronic measurements.
What are some applications of flexible electronic devices?
I’ll give you some examples that we have worked on and that are still a matter of very active investigation. One of the applications is transparent conductive films like the ones used in mobile phone screens. Because these materials are thinner than what is currently used in most phones, they are much more transparent. That translates into screens that are brighter or more efficient in their battery use.
Flexible electronics can also be used to make whole devices. For example, we’ve demonstrated a flexible radio, where you have a piece of plastic, you have a speaker on that piece of plastic, and that piece of plastic can receive signals – let’s say music signals – wirelessly and play them. So you can imagine having a foldable, flexible radio that you can take with you and unfold when you go to the beach.
Another application is graphene tattoos, where you put the tattoo on the skin, use it to record your vital signals, and then convey that information to an app on a mobile phone. A system like that can record your pulse, your heartbeat, your blood pressure and other information for health. We’re working on a system for continuous monitoring of blood pressure, which is very important for many diagnostic reasons in medicine.
You mentioned making a radio from flexible electronic components. More recently, you’ve been developing flexible memory components called atomristors. Could you explain what they are?
Of all the vast array of electronic components available today, memory or information storage components are the most important. These are the components that store information in binary code – ones and zeros – and those ones and zeros can represent any kind of data, from videos to images and so on. That’s why, when you buy a smartphone, if you get 512 gigabytes instead of 256 gigabytes of memory, it’s going to cost you significantly more money.
Because memory is so important, we knew that if we wanted to make a complete system of flexible electronics, we needed to have a flexible memory, too. And in order to make a flexible memory, you need materials that are themselves flexible, which implies that they must be very thin.
In this sense, 2D materials are the best class of flexible materials because they are the thinnest materials that exist in nature. Our atomristors are atomically thin, less than one nanometre thick, and you can make them from molybdenum disulfide, which is a common 2D material. And that can lead to flexible memories. We made that discovery a few years ago and we continue to advance it today.
Your most recent work is an extension of that project, but it involves a different 2D material, hexagonal boron nitride (hBN). Can you tell us more about that?
We were challenged by some of our sponsors to consider what other applications, in addition to information storage, could be served by our new memory technology. That led us to explore hexagonal boron nitride, which has the distinction of being the thinnest insulator on earth. We found out that, not only is it good as a memory, but it can also be used as a radio-frequency (RF) switch.
At the most basic level, a switch is a component that toggles between states, such as the different bands of communication we have on our mobile networks. For example, for 5G there are many, many bands: LTE, 4G, 3G, 2G, Bluetooth and so on. That means that RF switches need to be able to toggle rapidly between these bands, and ideally, they need to do that without consuming significant energy.
Our present switching technologies are based on transistors, but transistor switches consume a lot of energy. Even when they’re not toggling or switching, the standby energy to keep them operational is substantial. A memory switch like the kind we have been working on and developing is different, because its standby consumption of energy is zero. That would extend the battery life on your device significantly, and switches of this type are also much faster – they can handle not only 5G data but even 6G, which is 100 GB per second. For streaming applications, those speeds are unheard-of with current technologies.
Imagine having a foldable, flexible radio that you can take with you and unfold when you go to the beach
There was a lot of hype about graphene when it was first isolated. Why has it taken so long to exploit its electronic properties in a commercial device?
If you look at the history of technology, normally it takes 20 to 30 years to take an idea all the way from concept to product. Graphene has really only been in development for 10 years, and 2D materials, in general, a few years less than that. The technology is still at a nascent stage.
Nonetheless, several applications (including some that we have worked on) are on the market today. I mentioned graphene-based transparent conductive films earlier, and these are a real technology – you can buy them on certain smartphones in Asia, and the manufacturers have sold those phones in a reasonable volume. Some of our esteemed colleagues have also commercialized graphene sensor technology; I know there’s a start-up company in San Diego, California, called Cardea Bio that sells monolayer graphene biosensors.
There are also many other applications for graphene composites. An example is the packaging of smartphones, where composites help spread the heat around the back of the phone. And there are some consumer applications too, like using graphene to reinforce sportswear and the frames of bicycles so they are lighter and stronger.
What do you think the future holds for electronic devices made from 2D materials?
I think the future is brighter than it was a few years ago. The reason I say that is because several major semiconductor firms – including TSMC, Intel and some European companies – have invested a huge amount in terms of infrastructure, talent and R&D funds to try to integrate these materials into silicon-based semiconductor technology. Based on these trends from well-known, leading companies, I think we’ll see more 2D materials in electronic devices in the future.
However, as an addendum to that, when you consider the 2D materials that we’ve been working on, and many others across the world that have enjoyed billions of dollars in R&D funding, there’s a huge number of possibilities out there. It’s not one or two or five or a dozen materials. We’re talking about a thousand materials, and there’s a lot in this landscape that is undiscovered from a basic physics and science point of view. So even though there’s a lot of pressure for development and applications, there’s still a lot of basic science that we don’t know because there’s just so many materials to investigate.