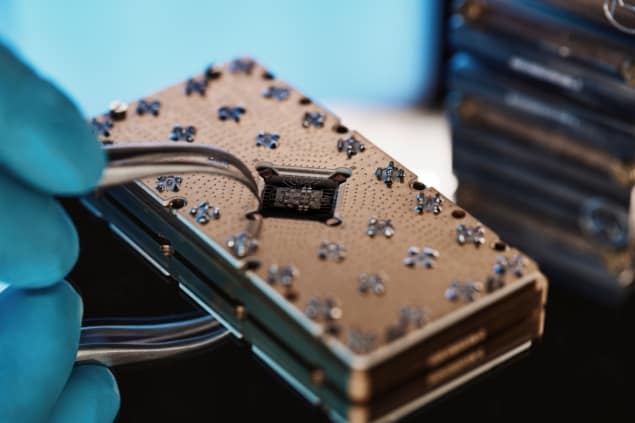
Quantum computers could surpass conventional computing in essential tasks, but they are prone to errors that ultimately lead to the loss of quantum information, limiting today’s quantum devices. Therefore, to achieve large-scale quantum information processors, scientists need to develop and implement strategies for correcting quantum errors.
Researchers at the Paris-based quantum computing firm Alice & Bob, together with colleagues at France’s ENS–PSL and ENS de Lyon, have now made significant strides towards a solution by enhancing the stability and control of so-called cat qubits. Named after Erwin Schrödinger’s famous thought experiment, these quantum bits use coherent states of a quantum resonator as their logical states. Cat qubits are promising for quantum error correction because they are constructed from coherent states, which make them intrinsically robust against certain types of errors from the environment.
A new measurement protocol
Quantum bits suffer from two types of errors: phase flips and bit flips. In quantum computing, a bit flip is an error that changes the state of a qubit from |0⟩ to |1⟩ or vice versa, analogous to flipping a classical bit from 0 to 1. A phase flip, on the other hand, is an error that alters the relative phase between the |0⟩ and |1⟩ components of a qubit’s superposition state. Cat qubits can be stabilized against bit-flip errors by coupling the qubit to an environment that preferentially exchanges pairs of photons with the system. This autonomously counteracts the effects of some errors that generate bit-flips and ensures that the quantum state remains within the desired error-corrected subspace. However, the challenge of quantum error correction is not just about stabilizing qubits. It is also about controlling them without breaking the mechanisms that keep them stable.
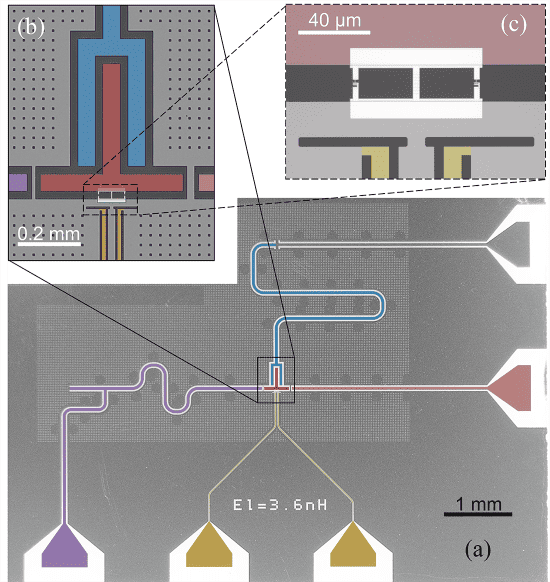
In the first of a pair of studies posted on the arXiv preprint server, and not yet peer-reviewed, researchers at Alice & Bob, ENS-PSL and ENS de Lyon found a way of increasing the bit-flip time to more than 10 seconds – four orders of magnitude longer than previous cat-qubit implementations – while still fully controlling the cat qubit. They achieved this by introducing a readout protocol that does not compromise bit-flip protection in their cat qubit, which consists of a quantum superposition of two classical quantum states trapped in a superconducting quantum resonator on a chip. Crucially, the new measurement scheme they devised for reading out and controlling these qubit states does not rely on additional physical control elements, which previously limited the achievable bit-flip times.
Previous experiment designs used a superconducting transmon – a two-level quantum element – to control and read out the state of the cat qubit. Here, the researchers devised a new readout and control scheme that uses the same auxiliary resonator that provides the two-photon stabilization mechanism for the cat qubit. As part of this scheme, they implemented a so-called holonomic gate that transforms the parity of the quantum state to the number of photons in the resonator. The photon number parity is a characteristic property of the cat qubit: an equal superposition of the two coherent states contains only superpositions of even photon numbers, whereas the same superposition but with a minus sign contains only superpositions of odd photon numbers. The parity therefore provides information about what state the quantum system is in.
Redesigning the stabilization of cat qubits
The Alice & Bob team prepared and imaged quantum superposition states while also controlling the phase of these superpositions and maintaining a bit-flip time of over 10 seconds and a phase-flip time longer than 490 ns. Fully realizing a large-scale error-corrected quantum computer based on cat qubits will, however, require not only good control and fast readout, but also a means of ensuring the cat qubit remains stable for long enough to perform computations. Researchers from Alice & Bob and ENS de Lyon addressed this important and challenging task in the second study.
To realize a stabilized cat qubit, the system can be driven by a two-photon process that injects pairs of photons while dissipating only two photons at once. This is usually done by coupling the cat qubit to an auxiliary resonator and pumping an element called an asymmetrically-threaded-SQUID (ATS) with precisely tuned microwave pulses. This approach, however, poses significant drawbacks, such as heat buildup, activation of unwanted processes, and the necessity of bulky microwave electronics.

To mitigate these problems, the researchers redesigned the two-photon dissipation mechanism so that it does not require such an additional pump. Instead of an ATS, they implemented the cat qubit in a superconducting oscillator mode coupled to a lossy auxiliary mode via a nonlinear element consisting of multiple Josephson junctions. The Josephson element serves as a “mixer” that makes it possible to exactly match the energy of two cat qubit photons to that of one photon in the auxiliary resonator. As a result, in this so-called autoparametric process, pairs of photons in the cat qubit resonator are transformed into a single photon of the buffer mode without the need for any additional microwave pump.
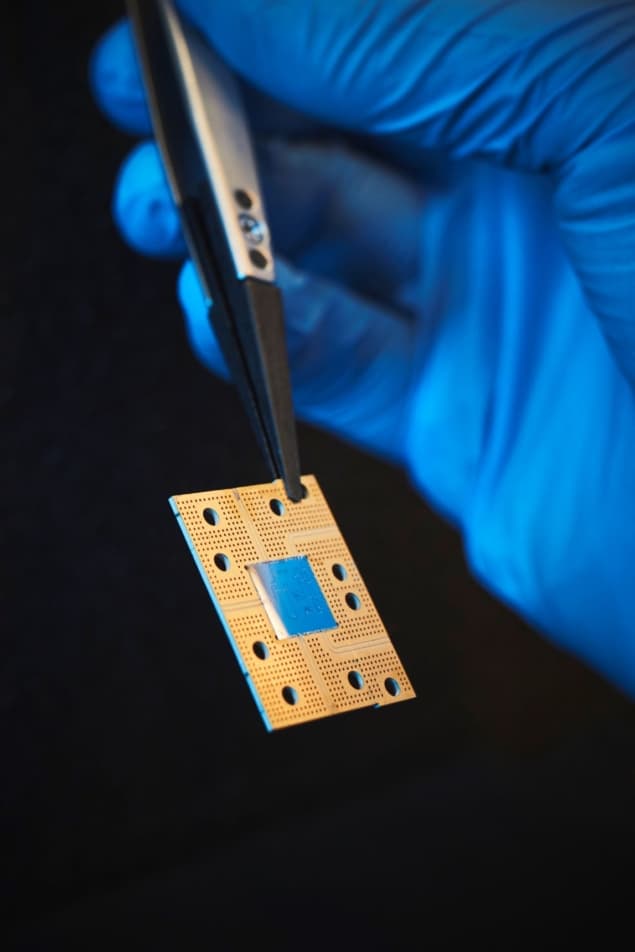
By designing a superconducting circuit with a symmetric structure, the team was able to couple a high-quality resonator with a low-quality one through the same Josephson element. They thereby increased the two-photon dissipation rate by a factor of 10 compared to previous results, with a bit-flip time approaching one second – in this case limited by the transmon. A high two-photon dissipation rate is needed for fast qubit manipulation and short error correction cycles. These are crucial for correcting the remaining phase-flip errors in a repetition code of cat qubits.
Future applications with cat qubits
Gerhard Kirchmair, a physicist at the Institute of Quantum Optics and Quantum Information in Innsbruck, Austria, who was not involved in either study, says that both works describe important steps towards realizing a fully error-corrected qubit. “These are the next steps towards full-fledged error correction,” Kirchmair says. “They clearly demonstrate that it is possible to achieve exponential protection against bit flips in these systems, which demonstrates that this approach is viable to realize full quantum error correction.”
The researchers acknowledge that significant obstacles remain. Because the accuracy of readout using the holonomic gate protocol was rather limited, they want to find ways to improve it. Demonstrating gates involving multiple cat qubits and checking whether the inherent bit-flip protection remains will be another important step. Furthermore, with the new autoparametric device setup to exchange pairs of photons, Alice & Bob co-founder Raphaël Lescanne anticipates being able to stabilize a cat qubit using four different coherent states instead of only two. “Our goal is to use the unprecedented nonlinear coupling strength to stabilize a four-component cat-qubit, which would offer in situ phase-flip error protection along with bit-flip error protection,” Lescanne says.
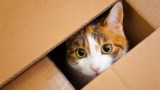
Why Alice & Bob are making cat qubits, IOP calls for action on net-zero target
Kirchmair believes these results pave the way for more elaborate error correction schemes relying on these heavily noise-biased qubits, where the bit-flip rate is much lower than the remaining phase flip rate. “Next steps will be scaling this system to also correct for phase flips thus realizing a fully error-corrected qubit,” Kirchmair tells Physics World. “One could even imagine combining both approaches in one system to make the best of both results and improve the bit flip times even further.”