The observation of baryons containing two charm quarks in a fixed-target experiment at Fermilab has surprised and puzzled the high-energy-physics community. Why do different doubly charmed baryons have such different masses?
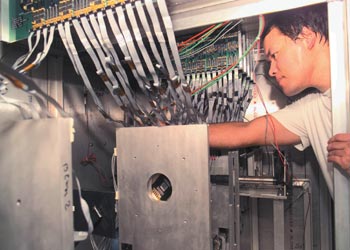
Particle physicists from all over the world met in Amsterdam recently for the International Conference on High Energy Physics (ICHEP), the flagship conference of the field. One result that stood out at the conference came not from the big collider collaborations working at the highest energies, or even from the new “B factories” that explore charge-parity violation, but from SELEX – an experiment at Fermilab in the US. In the SELEX experiment a 600 GeV particle beam strikes a fixed target made of copper or diamond foil to produce interactions of relatively modest energy.
Fixed-target experiments like SELEX have long been the poor cousins of particle physics, but in Amsterdam, James Russ of Carnegie Mellon University in Pittsburgh, the co-spokesman of the SELEX collaboration, presented the first evidence for doubly charmed baryons – particles that contain two charm quarks ( ICHEP:paper HQ-6-5).
Baryon basics
We are all well acquainted with the two most basic baryons, the proton and the neutron, and these make up the majority of the matter we see around us. However, there are many other baryons, such as Δ++, Σ+, Ξ0 and Ω– to name just four. Although all these baryons are incredibly tiny, to a particle physicist they are a big complicated mess of quarks whizzing around in a sea of gluons.
It was the quark model that brought some order to the chaos of the “zoo” of particles discovered in the 1950s and later formed the basis of the Standard Model of particle physics. In the Standard Model, all baryons contain three constituent quarks and, similarly, any combination of three quarks can form a baryon. Only three types of quark – the up (u), the down (d) and the strange (s) quarks – were needed to explain the particles known in the 1950s.
However, all quarks also have an intrinsic angular momentum or “spin” that is equal to 1/2, and this means that baryons can also exist in excited states. A proton, for instance, contains two up quarks and one down quark, and has an overall spin of 1/2 because two of the spins essentially cancel each other out. However, if all three spins point in the same direction, an excited state known as a Δ+ state is created (see figure 1). Many of these excited states are short-lived, which means, according to the Heisenberg uncertainty principle, that they have ill-defined masses. Since their mass is not well defined, these states are not graced by the description “particle” but instead are known as “resonances”.
In 1974 evidence for a fourth quark, the charm quark (c), was found. It immediately became clear that there must exist a whole family of charmed baryons, each containing a single charm quark and two “light” quarks (as the up, down and strange quarks are collectively known). The dynamics of charmed baryons are very appealing from a theoretical standpoint. The charm quark stays effectively at rest inside the baryon because it is much more massive than the up, down and strange quarks. The two light quarks can be considered as a “di-quark” pair and they bounce around inside the baryon at high speed.
Simply charming
Although still complicated, charmed baryons are simpler to analyse theoretically than light baryons because one of the constituents (the charm quark) is effectively at rest. A charmed baryon is in many ways analogous to a hydrogen atom, which consists of a proton, which is heavy, and a much lighter electron. By finding the energies of the excited states of a hydrogen atom, we can get an insight into the electromagnetic forces that bind it together. Similarly, the masses of the excited states of charmed baryons can provide us with information about the strong force that holds the quarks together.
The first charmed baryons were found in the late 1970s and 22 different states have now been catalogued. Most of these states were discovered at fixed-target experiments similar to SELEX and at electron-position colliders working at energies that are now considered rather modest. In particular, the CLEO experiment at the Cornell Electron Storage Ring (CESR) in upstate New York has discovered more than half of the known charmed-baryon states – the most recent being a charmed baryon with a mass that is more than 25% higher than that of the ground state, yet stable enough to be well defined (Phys. Rev. Lett. 86 4479).
The properties of charmed baryons are complicated but orderly and comprehensible. The discovery of doubly charmed baryons opens up the door to a whole new spectroscopy, complementary in nature to the one already studied. In this new regime there will be a heavy di-quark, effectively at rest, with a single light quark buzzing around inside the baryon.
A double surprise
Particle theorists enthusiastically use models to predict the masses of the states they know must exist and then wait for the experiments to confirm or reject their models. However, the claim that doubly charmed baryons have been discovered has created as much controversy as it has excitement. It is not that anyone doubts that they must exist, or that they should live briefly and then decay to, among other things, a singly charmed baryon, a kaon and a pion, as has been observed by SELEX. (Kaons and pions are examples of particles known as mesons, which contain a quark and an antiquark.)
However, there are aspects of the new results that are surprising: indeed, some physicists say they are too surprising to be believed. First is the issue of mass. The SELEX group believes that it has seen the signatures of two doubly charmed baryons – one containing two charm quarks and an up quark, Ξ++, and the other containing two charm quarks and a down quark, Ξ+. Although both masses reported by the SELEX team are quite reasonable in their own right, the difference between the two (about 2% of the total) is amazingly large.
The up and down quark are very similar, and add essentially identical mass to the system, and so the masses of the two new doubly charmed baryons should only differ by a tiny amount. Maybe it is this problem that has led the SELEX collaboration to submit the discovery of only one of the particles – the Ξ+ (ccd) baryon – for publication (M Mattson et al. 2002 ArXiv.org/abs/hep-ex/0208014).
The second amazing fact is that enough doubly charmed baryons were produced by the collisions for the SELEX team to have found them. They estimate that 40% of the singly charmed baryons they see are the result of the decay of doubly charmed baryons. However, this is one or two orders of magnitude greater than would be expected from simple hand-waving arguments.
Is this evidence of a new production mechanism that had not previously been considered? The FOCUS experiment at Fermilab has also studied charmed baryons in a beam-dump experiment. At the Amsterdam conference the FOCUS team presented null results in a search for doubly charmed baryons in 21 different decay chains. The CLEO collaboration has not yet presented any data on its search for doubly charmed baryons, but the researchers surely would have noticed if 40% of their charmed baryons were the decay products of ccu and ccd particles.
Unfortunately, it impossible for either the FOCUS or CLEO experiments to rule out the SELEX result because the processes by which the particles are made are different. Maybe there is something in the SELEX beam – a 600 GeV beam that contains both protons and strange baryons – that preferentially produces doubly charmed baryons. The situation is not likely to be completely clarified for some time.
The last of the singly charmed baryon ground states, the Ωc (css) baryon, was first reported in 1974. However, it was only in the last two years that results from CLEO, FOCUS and the BELLE experiment in Japan have converged to give consistent and universally accepted measurements of its mass. It could take many years of careful measurements and re-measurements before the doubly charmed signals can be verified and understood. These particles only live for about 10-13 seconds, but their study can occupy a lifetime.