Christoph Gerber, who co-invented the atomic force microscope, tells Matthew Chalmers how the AFM came about 30 years ago and why it continues to shape research at the nanoscale
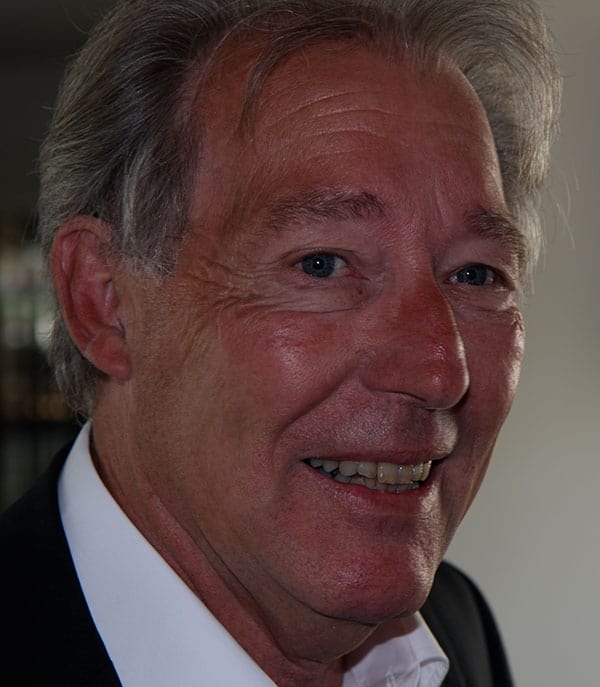
Where would nanotechnology be without the AFM?
Nanotechnology would be at a far less advanced stage had the scanning tunnelling microscope (STM) and atomic force microscope (AFM) not been invented. Seeing is believing; so when you can image individual atoms on a surface, it has a major impact. The STM took scientists into uncharted territory by offering the ability to image surfaces in 3D at the atomic scale, but the AFM opened up the field much further – from numerous applications in the semiconductor industry and surface science to biological and medical applications, to mention a few. We really couldn’t foresee this at the time.
Didn’t we already have atomic resolution with the electron microscope?
The breakthrough with STM and AFM was that you don’t need to have a vacuum to do experiments, and the techniques are not limited in spatial resolution by diffraction. The AFM is sensitive to a range of surface interactions that include chemical, mechanical, electrostatic, capillary, electromagnetic and other forces, and you can use them for different experiments. Recently at IBM Research Zurich, Leo Gross and Gerhard Meyer have been able to pick up a surface molecule with the tip and use it to image adsorbed molecules on a surface, thus revealing the chemical bonds present.
How did the AFM evolve from the STM?
The STM was invented in 1981 and five years later Gerd Binnig and Heinrich Rohrer shared the Nobel Prize for Physics for the work. Since the STM can only image electrical conducting surfaces, the question was, how can we image atomic detail in non-conductive surfaces? This idea was discussed at the first workshop devoted to the STM, which was held in Austria in 1985, when researchers began to confirm the atomic-scale 3D imaging reported by IBM three years earlier. Everyone present realized that something very special had happened: we were witnessing the beginning of nanoscience. At that time, Gerd and I were on sabbatical leave based in Cal Quate’s group at Stanford University and also IBM Research Almalden. Gerd suggested that it might be possible to measure the interactive forces, rather than the current, between tip and sample, and that perhaps we could do this with a cantilever. We did a rough calculation and realized that in order to get atomic resolution we needed to be able to detect forces at the level of 10–10 N or even 10–11 N!
How did you go about detecting such a tiny force?
I started to design a configuration of the first system. The heart of the device was a very thin gold foil just a few microns thick used as a cantilever. We took a fragment from a crushed diamond obtained from the stylus of a record player and glued it onto the cantilever to serve as a tip. Since the STM can measure displacements of 10–4 Å, it was the obvious choice to measure the tiny forces that deflected the cantilever. The device also had to be very rigid to minimize mechanical vibrations and prevent the tip from crashing into the surface. Within five months we had a working system. We did not get atomic resolution straight away but we were close enough to submit a journal paper. Within a year we had a more advanced instrument based on a batch-fabricated silicon cantilever that showed atomic resolution for the first time on a graphite surface. Atomic resolution on non-conductive surfaces took researchers a further eight years.
How did the community respond to the invention?
The AFM was welcomed very favourably by the scientific community – unlike the STM, which was initially thought to be too exotic and was not expected to work. As soon as we had presented the AFM, people immediately started to improve on our ideas – for instance using lasers to detect the cantilever motion – and by the late 1980s it had really taken off.
What has been the biggest impact of AFM?
The AFM has become the most versatile nano-toolkit we have, and it has stepped out into so many other disciplines operating over a vast temperature range and essentially in all environments. To date there are more than 350,000 publications on their AFM, more than 700 patents and the original AFM paper (Phys. Rev. Lett. 56 930) has been cited more than 9000 times. The AFM is also easily combined with other technologies to make it even more powerful. One impressive recent advance came in biology, where Toshio Ando and colleagues at Kanazawa University in Japan developed a high-speed AFM capable of imaging cellular machinery dynamically in real time. It took the group some 15 years to achieve this goal, which would be almost impossible to undertake today with current funding philosophies. Back in the 1980s we were just asked to be original, which was the culture of IBM at that time.
Is it right that the Nobel prize was only credited to the STM?
Yes. The AFM is not as closely connected to the STM as is often claimed. The STM will always be in an academic setting, for example, whereas the AFM has branched out into numerous disciplines. To a certain extent, the AFM has surpassed the STM because it is a tool that is applicable to so many different fields.
Where might the AFM take us next?
As an example, the original AFM is part of an exhibition at the Science Museum in London called “The making of the modern world”. The nearby Apollo capsule is therefore in good company because an AFM has already been sent to the Martian surface and one is currently on board the European Rosetta mission to investigate stardust on the nanoscale. It seems not even the sky is the limit for AFM technology.