Complexity science – which describes phenomena such as collective and emergent behaviour – is the focus of a new centre where researchers are examining everything from the spread of influenza to what a healthy heartbeat looks like. Sergio de Régules reports
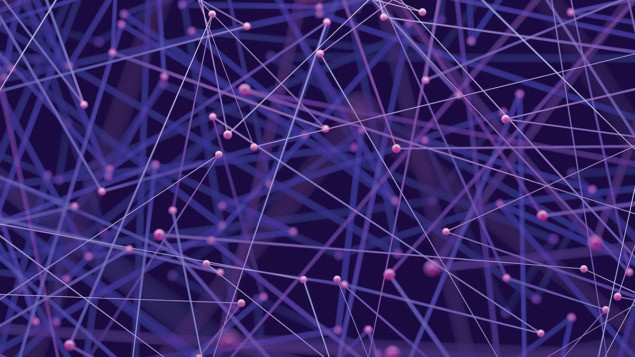
It is a crisp winter day on the Mexico City campus of the National Autonomous University of Mexico (UNAM). A clear blue sky creates a pleasing contrast with the dark rocks on which the entire campus, as well as the surrounding Pedregal residential area, is built. The black and grey lava fields were spewed 1700 years ago by the neighbouring Xitle volcano, and as I make my way towards a white, two-storey building – all concrete, glass and steel – it looks under the dazzling Sun like a UFO has just landed on the ancient volcanic rock.
The starship is the new Centre for Complexity Science (Centro de Ciencias de la Complejidad, or C3), and as for its approach it might as well have come from another planet. The tradition at UNAM is for staff to have tenured faculty positions with little sideways mobility; indeed, scientists can end up staying in the same research institution and keeping the same office for decades. The C3 adopts a different model – that of the Santa Fe Institute in New Mexico, which is the world-leading powerhouse of complexity. Temporary positions and offices are offered to visiting professors, in fields ranging from physics and computer science, to history and anthropology. “There are no nameplates on the doors,” says C3 co-ordinator Alejandro Frank, a nuclear physicist turned complexologist, who about a decade ago decided to break free of the confinement of the nucleus. “For years I was stuck at 10–15 m,” he says. Complexity offered a portal to a wider variety of scales.
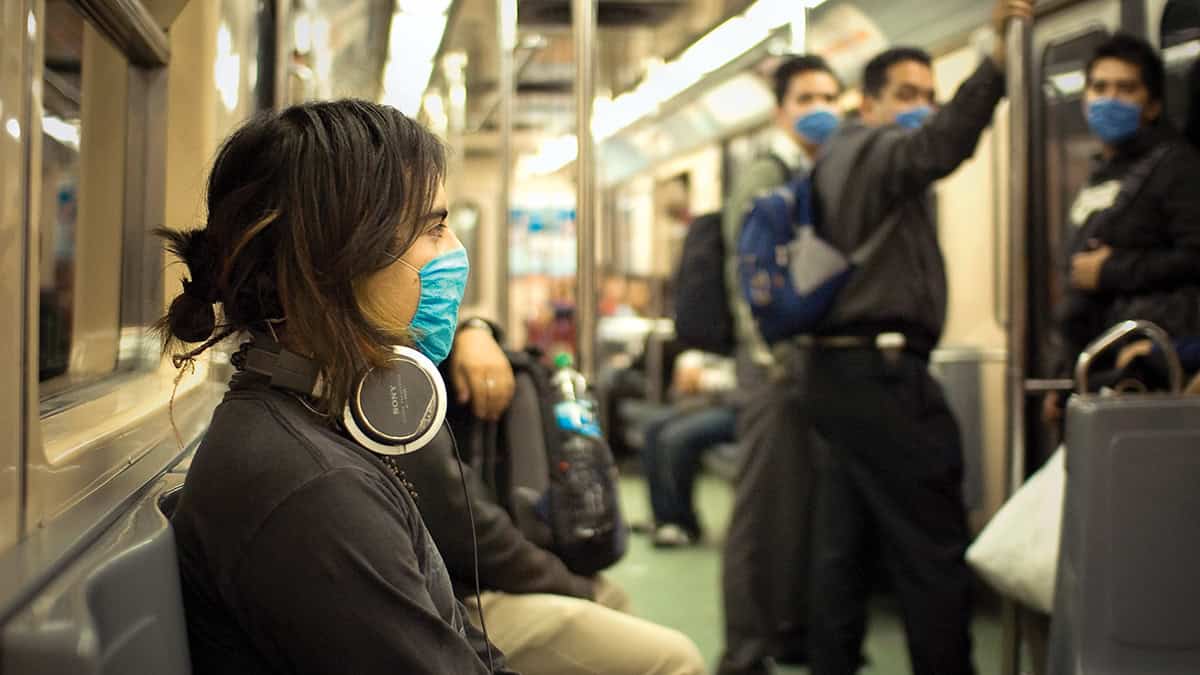
The latest addition to UNAM’s research community is all about flow and adaptability, as opposed to the rigidity common in other scientific fields, which Frank says he wants to avoid. “We need to be able to summon multidisciplinary groups of academics in short order to respond to crises,” he says, recalling the H1N1 swine influenza pandemic of 2009 that brought Mexico to a standstill when health officials recommended that people stay at home to avoid contagion. Frank feels that the scientific community’s response to the crisis might have been nimbler had information flowed more freely between scientists in different fields and institutions. The C3’s flexibility and inclusiveness are intended to change that.
Symbolic location
While the C3 building is new, the centre itself is more mature, having already existed virtually for 10 years as a research community that began with some 25 members. Meetings, when necessary, were held in rented quarters at UNAM’s Faculty of Engineering, where the complexity theorists were eyed with suspicion. Eventually, Frank managed to get the attention of the then university chancellor José Narro, who offered to underwrite the construction of a purpose-built facility.
Once the funding had been agreed, the next step was for Frank to find an appropriate spot on campus for the building. The Pedregal lava fields are home to diverse fauna and flora, and large swathes of university-owned land are protected by the UNAM Wildlife Reserve. Frank’s search around the campus for land on which to build the new centre was therefore limited to non-reserve grounds. He found a spot between the Universum Science Museum, the performing arts complex and the humanities research district. “That is the place I wanted,” says Frank, “at the crossroads between science, the arts and the humanities.” He was delighted to find this symbolic location given how multidisciplinary complexity is.
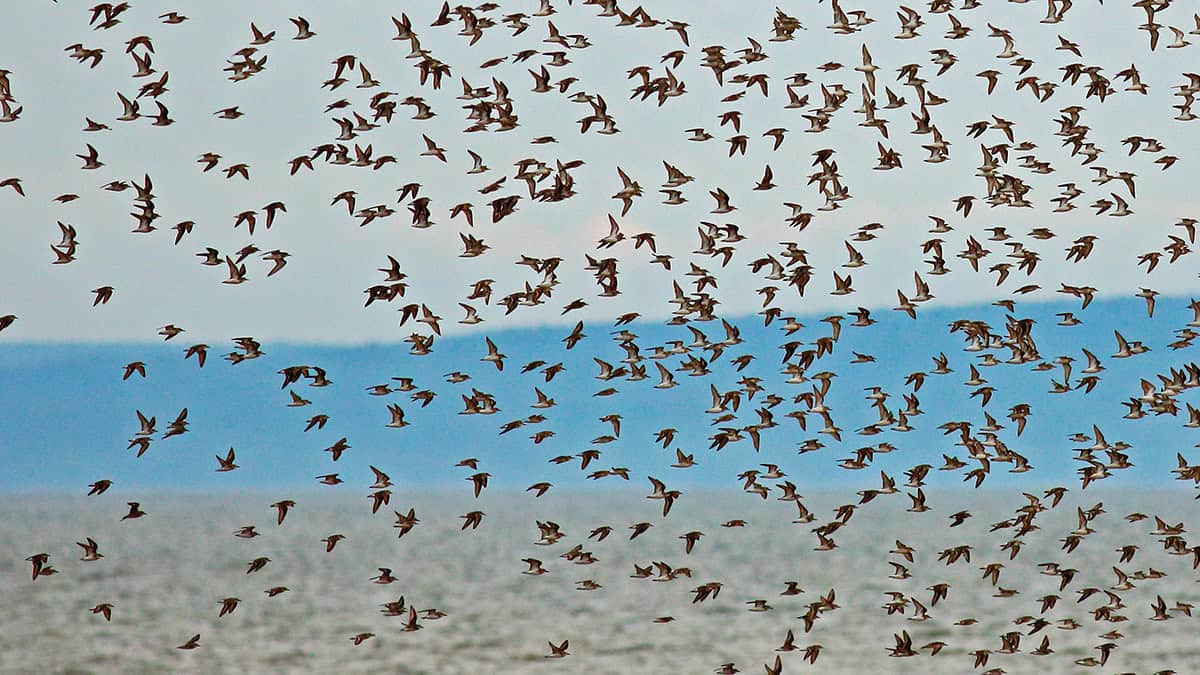
There is a reason for this multi-faceted nature. “What is the difference between regular scientific problems and complex problems?” asks Frank rhetorically. “It’s like comparing the nucleus of an atom with a pond in the jungle.” While the nucleus is relatively simple and everything in it takes place at the same scale, in the pond entities at different scales are interacting – plankton, mosquitoes, fish, insects and mammals, for example. If some toxic matter were dumped into the pond, it might only affect the plankton directly; however, changes to the plankton would in turn cascade to affect the wildlife and the whole system would be altered. “The essence of a complex system is the connection between these different scales, so you need specialists in each,” says Frank. “Multidisciplinarity emerges naturally from the multi-scaled nature of the problems.”
The new building opened in October 2015. The complexity nomads finally had a home. During the ceremony, chancellor Narro joked: “I think Alejandro Frank misunderstood me when I said it was time to go from the virtual to the concrete!”
Collective phenomena
Since the early 20th century, scientists have increasingly recognized that real-world problems, as opposed to textbook problems, are often not amenable to the spherical-cow reductionism still emphasized in traditional science education (the joke is that a physicist begins a calculation by saying “consider a spherical cow”). A good example occurred late last year, when a tropical storm approaching the shores of western Mexico turned overnight into a category 5 hurricane that threatened to devastate the towns of Manzanillo and Puerto Vallarta. A reductionist approach to the problem of understanding, predicting and controlling hurricanes might begin by treating the storm as an isolated bunch of gas and water molecules in collision, perhaps applying statistical mechanics to allow for the fact that there really are a lot of molecules. The complications of mass and energy exchange with the environment could be worked in later as perturbations. However, no amount of persistence along this path will explain the surprisingly explosive growth of Hurricane Patricia and its subsequent quick demise, to say nothing of its erratic trajectory. A hurricane is more than the sum of its molecules. It is not merely complicated: it is complex.
In a recent paper in PLOS ONE (10.1371/journal.pone.0130751), a C3 team characterizes complex systems as “those in which the agents or elements that compose the system interact nonlinearly and in such a convoluted way that it is impossible to describe the behaviour of the system in terms of the simpler behaviour of its components”. This is a good description for many phenomena: the weather, the Internet, market crashes, turf wars between gangs, epidemics, social organization in humans and in animals, and even city traffic. They all exhibit an interplay between their component parts that goes far beyond the relatively simple, large-number behaviour statistical mechanics describes.
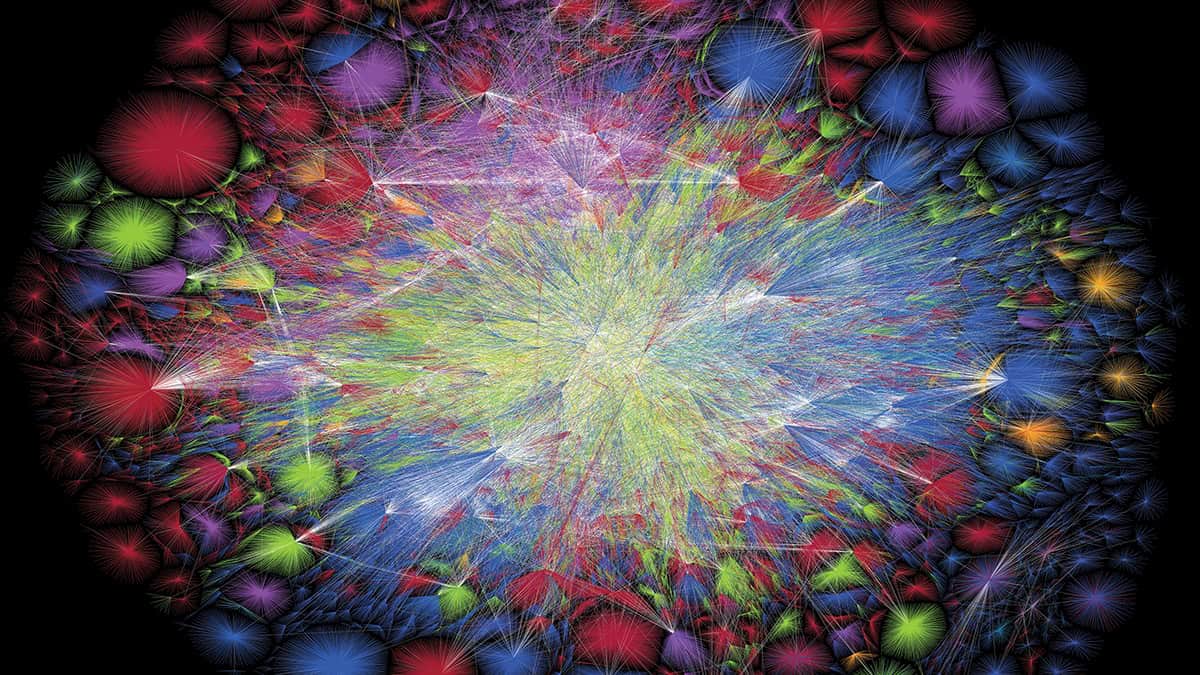
This manifestation of collective phenomena that are impossible to predict from the individual behaviour of the parts – such as the direction in which a swarm will next turn – is called emergence. While reductionism fails when things get convoluted and nonlinear, complexity theory can describe these simple universal patterns of a higher order. An ocean wave is an emergent property of water molecules. Thoughts emerge from the firing of millions of neurons. Even the economy emerges from our individual financial decisions. Complex systems naturally self-organize – a bit like an ant colony (or a research centre), where order does not come from any one individual actually calling the shots, but materializes from the synergy of the group.
Close to the edge
Another idea from complexity theory is that the really interesting phenomena happen at the boundary between order and chaos. Science journalist John Horgan explains in an article published in June 1995 in Scientific American (272 32): “The basic idea is that nothing novel can emerge from systems with high degrees of order and stability, such as crystals. On the other hand, completely chaotic systems, such as turbulent fluids or heated gases, are too formless. Truly complex things – amoebae, bond traders and the like – appear at the border between rigid order and randomness.” This means there is a Goldilocks zone with just the right mix of organization and turmoil. Systems poised in this dynamical sweet spot are said to be “critical” in much the same way as water near the freezing point.
Back at the C3, scientists are bringing this concept to bear on cardiac disease and diabetes, two important health issues in Mexico. “All living things tend to criticality. This is their equilibrium,” says Frank, paraphrasing Stuart Kauffman, one of the founding fathers of the field.
As to why criticality can be a good for living things, consider the heart. It has two opposing functions: body tissues need nutrients constantly, so the heart better be robust and dependable, which requires order and rigidity. But the environment is constantly changing, so the heart also has to be adaptable. “It’s not a Swiss watch. It needs flexibility,” says Frank, who, together with others at C3, is working to identify early warnings of imminent catastrophe in everything from heart disease and epilepsy, to volcanic eruptions and earthquakes, which are also a concern in Mexico.
The heart’s criticality, or lack thereof, can be seen in time series plots of the heartbeat. Any cardiologist worth their salt can glean lots of information about a patient’s heart from a regular electrocardiogram (ECG), which plots cardiac electrical activity against time. Now researchers are looking at graphs not of the oomph of each beat, but of the heart’s changing rhythm. This is easily visualized in time series, in which what is plotted are changes in the interval between successive heartbeats. Time series are a measure of how well the cardiac muscle beats time – so too are their power spectra, which do to a graph what a prism does to light: separate it into its component frequencies.
“I once showed these graphs to a bunch of cardiologists,” recounts Frank, who presented three different versions: one was flat, another wandered up and down randomly, and yet another fluttered gently up and down like a butterfly. “I asked which of them belongs to a healthy patient. They had no idea,” he says. It turns out that a rigid, metronome-like beat is a sign of disease, while a healthy heart beats to a jazzier, more free-flowing rhythm. “The flat graph belonged to a patient with heart failure; the heartbeat was too constant, [with] no flexibility to adapt. The randomly varying graph indicated fibrillation: the patient was on the brink of death. The fluttering graph showed a heart that was neither too rigid, nor too chaotic” – a heart snuggled in the critical sweet spot.
“Living things tend naturally to criticality,” Frank insists, and deviations from it signal that something has gone awry. “We have proposed a project with the National Institute of Medical Science and Nutrition to gather data on patients about to undergo different procedures (stent surgery, or after a heart attack), to see if the procedure restores criticality.” (For more on using physics techniques to analyse heart rates, see “Revealing the network within”, February pp29–31).
In the paper published in PLOS ONE (of which Frank is a co-author), the team expresses confidence in the wide applicability of these ideas. “All these transitions display characteristic signals that in principle are independent of the particularities of the system. In other words, the dynamics of the systems near critical points exhibit universal properties.” And these properties could be rallied to identify warning signs of impending heart attacks, earthquakes, volcanic eruptions, and even diseases such as cancer and diabetes.
Building for complexity
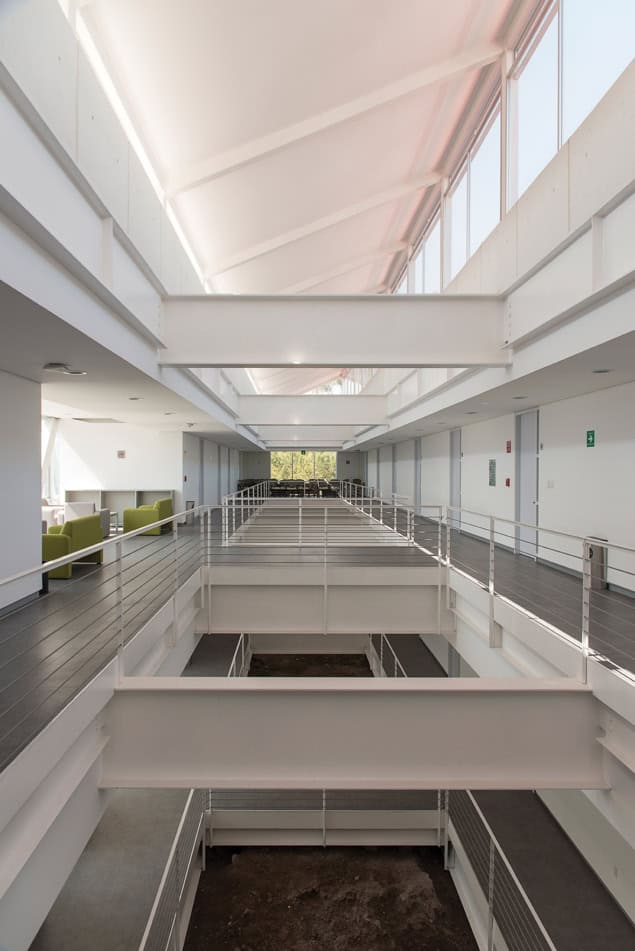
It is perhaps appropriate that the very building that houses the C3 should embody the principles and philosophy of complexity science. Architects Susana García Fuertes and Francisco Serrano designed a dynamic workspace with reconfigurable walls so that spaces can be easily adapted to the needs of the projects being pursued at any moment. Following the trend of “social buildings” that encourage occupants to interact, García Fuertes and Serrano distributed cosy sitting areas for spontaneous meetings throughout the C3. The spirit of collaboration is reflected in the openness created by the almost complete absence of columns and load-bearing walls. This is thanks to a visible exoskeleton of steel cross beams supporting the structure.
Sustainability was another concern. “It was important that the building not touch the ground, that it respect this wonderful, rocky soil,” says García Fuertes. “So there are few points of contact with the ground and the structure seems to float.” Hence the impression of a spaceship that has just touched down.
The inner walls of the building are coated with a special paint that effectively turns them into giant whiteboards. Researchers chatting in the corridors can use markers to draw sketches, write equations or generally adorn the walls with whatever strikes their fancy. “We want the decoration to emerge,” says Frank. At the time of writing, the walls of the UFO remain a blank page, waiting for the first scribblings from the C3 community.