
Entangled particles – that is, those with quantum states that remain correlated regardless of the distance between them – are important for many quantum technologies. Devices called Cooper-pair splitters can, in principle, generate such entangled particles by separating the electrons that pair up within superconducting materials, but the process was considered too random and uncontrollable to be of practical use.
Physicists at Aalto University in Finland have now put forward a theoretical proposal indicating that these electron pairs could, in fact, be split on demand by applying time-dependent voltages to quantum dots placed on either side of a superconducting strip. The technique, which preserves the entangled state of the separated electrons, might aid the development of quantum computers that use entangled electrons as quantum bits (qubits).
When a conventional superconducting material is cooled to very low temperatures, the electrons within it overcome their mutual repulsion and pair up. These so-called Cooper pairs propagate through the material without any resistance. The paired-up electrons are naturally entangled, with spins that point in opposite directions. Extracting and separating these electron pairs while preserving their entanglement would be useful for a host of applications, including quantum computing, but doing this is no easy task.
In the latest work, which is detailed in Physical Review B, physicists led by theorist Christian Flindt propose a new way to operate a Cooper pair splitter. Their design consists of a superconducting strip that contains two electrodes and is coupled to two quantum dots (nanosized pieces of semiconducting material) on either side of the strip. When a voltage is applied to the electrodes, Cooper-paired electrons within the superconductor are drawn to the tip of the superconducting strip and become separated, with each quantum dot accommodating one separated electron at a time. These separated electrons can then be passed on through a nanowire.
Time-dependent voltages
The key to the team’s set-up is that the voltage applied to the electrode on one side of the strip varies in time such that exactly two Cooper pairs are split and ejected during each periodic oscillation. “In experiments so far, the applied voltages were kept constant,” Flindt explains. “In our proposal, we show how the splitting of Cooper pairs can be controlled with time-dependent voltages applied to the device.”
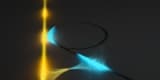
New device entangles free electrons with photons
Based on their calculations, Flindt and colleagues estimate that their Cooper-pair splitter could separate entangled electrons at a frequency in the gigahertz range. Most modern computers operate with clock cycles in this range, and for many quantum technologies it is important to have a similarly fast source of entangled particles. Indeed, combining several splitters together could help form the basis of a quantum computer that operates using entangled electrons, the team says.
Experimentalists invited to “pick up the baton”
The Aalto physicists decided to undertake their study because they realized that there was a need to control the splitting of Cooper pairs. Their biggest challenge was to figure out how to vary the voltages in time such that the Cooper pairs would be split on demand. Looking forward, they think it should be possible to realize their proposal experimentally and hope that experimentalists will “pick up the baton”.
“It would also be interesting to investigate how our on-demand Cooper pair splitter can be integrated into a larger quantum electronic circuit to develop quantum information processing,” Flindt tells Physics World.