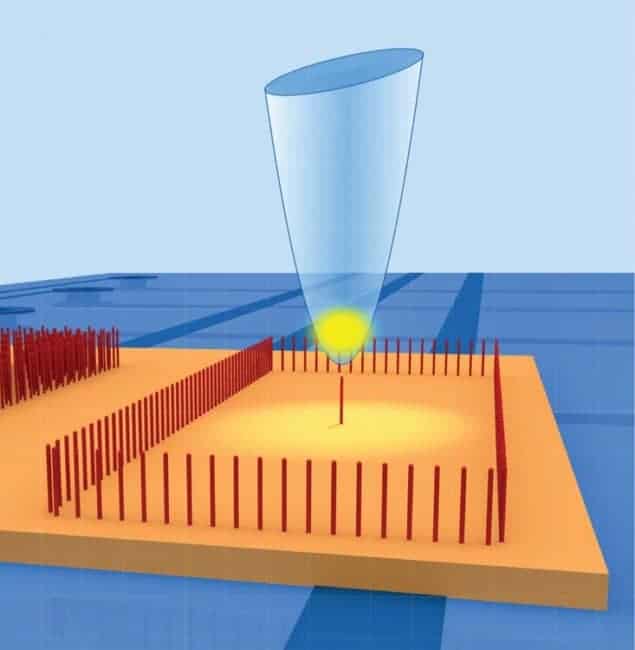
Physicists in Germany have invented a new kind of microscope that uses a gas of extremely cold atoms to map the surface of nanoscale structures. The researchers say that their device is complimentary to atomic-force microscopes (AFMs) and that they ultimately hope to create a probe with precision that is limited only by fundamental quantum uncertainties.
AFMs work by running a tiny tip attached to a cantilever arm along the surface that needs to be imaged. As the tip moves up and down in response to the changing contours below, the cantilever is deflected and these movements are measured by a laser beam that is bounced off the cantilever. Finally, a plot of the laser deflection against tip position generating a map of the surface.
The latest device, built by Andreas Günther, József Fortágh and colleagues at the University of Tübingen, uses a gas of cold atoms rather than a solid tip to map surface contours. Objects selected for imaging are mounted onto a chip, which is placed above a sapphire substrate. The object and substrate are then sandwiched between networks of gold wires that generate a 3D magnetic field. Suspended within the field is a cloud of rubidium atoms, thanks to the fact that rubidium is paramagnetic, and then the field is adjusted so as to lower the atoms just above the objects under study.
Atoms ejected
As the cloud is scanned along the chip, a laser beam is shone through the atoms and the absorption of the beam is measured. With the atoms held at a temperature of less than 1 µK and the objects existing at room temperature, any contact between the two will impart a huge energy to the atoms, causing them to be ejected from the cloud. So as the gas moves along the surface, any reduction in absorption reveals the atoms, leaving the gas and therefore the presence of a surface.
Günther and co-workers used the “cold-atom scanning-probe microscope” to image a single carbon nanotube, with a diameter of several tens of nanometres, surrounded by other nanotubes arranged in a rectangular shape. They carried out the observations in both contact mode, measuring the loss of atoms from the cloud, and in dynamic mode, in which variations in the oscillation frequency of the centre-of-mass of the cloud reflect the changing contour below. They also performed the imaging with the gas both as a classical, or “thermal”, entity, and as a “Bose–Einstein condensate”, which is formed when identical atoms with integer spin are cooled until all of the atoms occupy the same quantum state. They found that they could achieve higher spatial resolution when the atoms existed as a condensate, partly because this enabled them to make the cloud smaller.
The researchers point out that because the microscope cannot reveal details smaller than the scale of the atom cloud, its resolution is at the micron scale, 1000 times higher than that of an AFM whose tips consist of a single atom. But they believe that the two kinds of microscope are complimentary, with AFMs imaging details closer to the surface and the cold-atom device able to measure forces further from the surface. This, they say, could enable the measurement of magnetic fields above nanoelectronic components in order to characterize the current flow in these devices. Alternatively, the microscope could be used to characterize various properties such as van der Waals forces of carbon nanotubes when they are used in biosensors to capture certain biological molecules.
Exploiting quantum properties
The next major step in the development of the microscope, says Fortágh, is to try and exploit the potential of the gas as a Bose–Einstein condensate.” In this latest research we used the condensate to reduce the size of the cloud,” he says. “But we didn’t exploit the quantum mechanical properties of the condensate.” He wants to use the quantum probe to make quantum-limited measurements, which are never possible in a classical system because of decoherence. He estimates that further reductions in the size of the cloud over the next 5–10 years might bring the resolution of the new microscope close to that of an AFM.
Martin Breitschaft of the University of Augsburg in Germany describes the latest work as “very intriguing”. He points out that the research could lead to improved designs of tips used in conventional scanning probe microscopes. These probes are often made by carving them out of bulk materials in a time-consuming process where the structure of the resulting tips is often poorly known and sometimes dominated by impurities. “Because the probe in the latest work is built up artificially from scratch, this or similar techniques might eventually enable the systematic design of probes with predefined microscopic properties,” he said.
The research has been published online on Nature Nanotechnology.