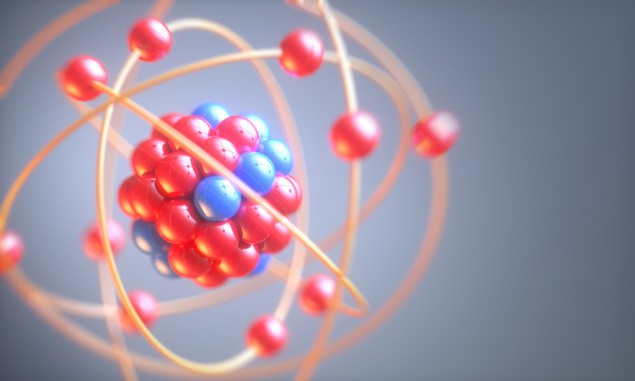
According to the classical model of nuclear structure, the internal structure of nucleons should not change if they are bound into atomic nuclei. But it was discovered 35 years ago that quarks inside free protons and neutrons behave differently to those bound into nuclei – and the cause has remained a mystery ever since. Now researchers have taken a significant step towards solving the puzzle by using two different types of scattering experiments to relate the strength of the effect to the number of high-momentum nucleon pairs in an atomic nucleus.
According to the standard model of particle physics, the energies binding quarks inside protons and neutrons are almost 100 times larger than the energies binding those nucleons inside nuclei. In the 1980s, therefore, researchers at the European Muon Collaboration (EMC) at CERN assumed they could safely speed up their nucleon-probing experiments by using iron nuclei containing many nucleons.
“They thought there was no way the relatively small binding energy of an iron nucleus would have any effect on the quarks,” explains nuclear physicist Axel Schmidt of Massachusetts Institute of Technology.” To their surprise, however, they found that the collision cross-section per nucleon was lower than expected, implying that the momentum distribution of the quarks had changed.
This has subsequently been confirmed in other experiments in other atoms: “Every single nucleus that’s subsequently been checked has some degree of this effect,” says Schmidt, “And the bigger the nucleus, the bigger the effect.”
Alternative theory challenges the mainstream
The cause of the so-called EMC effect remains unexplained, however. “Many theories have been rejected by experiment,” says Schmidt. “Now there are really only two surviving classes of theory. I would say the mainstream view is that something about the environment of the nucleus changes the quark distribution of all the constituent protons and neutrons.”
The other theory relates the effect to transitory correlations between nucleons, which lead them to have much higher momentum than other nucleons. “Perhaps 80% of the protons and neutrons are unchanged and 20% are changed dramatically and, when we measure the EMC effect, we’re measuring the aggregate,” he says.
Schmidt and colleagues from the CLAS collaboration, which is based at the Jefferson Laboratory in the US, decided to test this hypothesis. They reasoned that “if the effect is due to pairs, then every proton–neutron pair should be like every other – whether that pair is in a carbon or a lead nucleus,” says Schmidt.
The team found a neat way to directly measure both the proportion of high-momentum pairs in nuclei and their collision cross-sections at the same time. They irradiated targets of carbon-12, aluminium-27, iron-56 and lead-208 with high-energy electrons. Some of these electrons underwent quasi-elastic collisions with the nuclei, knocking out a proton or neutron but leaving the constituent quarks undisturbed. From these, the researchers could reconstruct the momentum of the nucleon at the moment of impact.
Other electrons underwent deep inelastic scattering, obliterating a nucleon and allowing the researchers to infer the average momentum distribution of the quarks inside the nucleons. Sure enough, the number of pairs in a nucleus was linearly proportional to the strength of the EMC effect, suggesting all pairs had the same effect.
Next, the researchers made a prediction. Protons are far more likely to form high-momentum pairs with neutrons, and vice versa. Therefore, in heavy, neutron-rich nuclei, protons are more likely to be paired at any instant, making their average momentum higher – a prediction that the CLAS collaboration confirmed last year. The researchers therefore calculated average EMC effects per proton and per neutron.

Protons go faster in neutron-rich nuclei
As predicted, the EMC effect per neutron stayed roughly constant at atomic masses beyond 12, but the EMC effect per proton continued to increase for all measured nuclei. “We think that this lends credence to the alternative hypothesis and suggests that we need to do follow-up experiments specifically to look at the quarks inside correlated nucleons, rather than just at the nucleus in aggregate,” says Schmidt. “There’s one we’ve just started and another scheduled for a few years from now.”
“As far as deep inelastic scattering results go, the mean-field hypothesis works probably as well as the short-range correlations hypothesis,” says Gerald Miller of the University of Washington at Seattle. “The fact that the researchers can independently measure the number of neutron–proton pairs interacting and establish a correlation is a big step. That said, it’s not quite nailed down yet because the alternative hypothesis has not yet been used to calculate this second set of kinematics. That presents a challenge that proponents of the alternative hypothesis need to meet.”
The research is published in Nature.