Scientists within the JEM-EUSO collaboration are exploiting innovative detector technologies in their quest to observe ultrahigh-energy cosmic rays from space
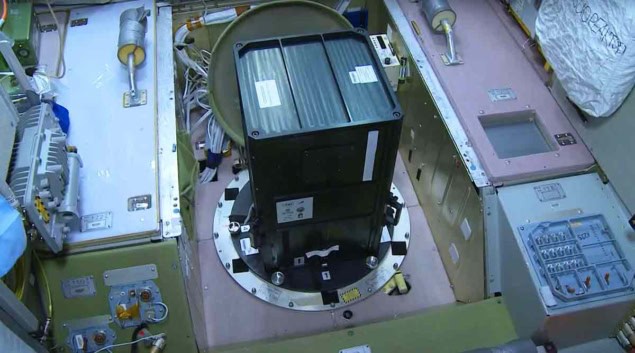
Physics at the extremes provides the raison d’être for the JEM-EUSO research collaboration – or, in long form, the Joint Exploratory Missions for Extreme Universe Space Observatory. Over the past 20 years or so, more than 300 scientists from 16 countries have been working collectively towards the JEM-EUSO end-game: the realization of a space-based, super-wide-field telescope that will scan the night sky and enable astrophysicists to understand the origin and nature of ultrahigh-energy cosmic rays (upwards of 5 x 1019 eV). In other words, JEM-EUSO promises to open a unique window into the Universe at energy regimes far beyond the current generation of man-made particle accelerators.
Looking at the sky from above
For context, cosmic rays are extraterrestrial particles comprising hydrogen nuclei (around 90% of the total) and helium nuclei (roughly 9%), with the remainder made up of heavier nuclei and electrons. Their energy range varies from about 109 to 1020 eV and beyond, while their flux is similarly spread across many orders of magnitude – ranging from 1 particle/m2 per second at low energies (around 109 eV) out to roughly 1 particle/km2 per century at extreme energies (around 1020 eV).
While it’s possible to detect cosmic rays directly at low-to-intermediate energies (up to 1015 eV), the flux of particles is so low at higher energies that indirect detection is necessary. In short, that means observing the interaction of cosmic rays (as well as neutrino decays) with the outer layers of the atmosphere, where they produce cascades of subatomic particles known as “extensive air showers” (or secondary cosmic rays).
With this in mind, the JEM-EUSO collaboration has rolled out an ambitious R&D programme over the past decade, with a series of pathfinder experiments geared towards technology validation ahead of future space-based missions (aboard orbiting satellites) to observe cosmic rays at their highest energies. Projects to date include ground-based installations like the EUSO-TA (deployed at the Telescope Array site in Utah, US); various stratospheric balloons (the most recent of which is EUSO-SPB2); and MINI-EUSO (Multiwavelength Imaging New Instrument for the Extreme Universe Space Observatory), a telescope that’s been observing the Earth from inside the International Space Station (ISS) since 2019.
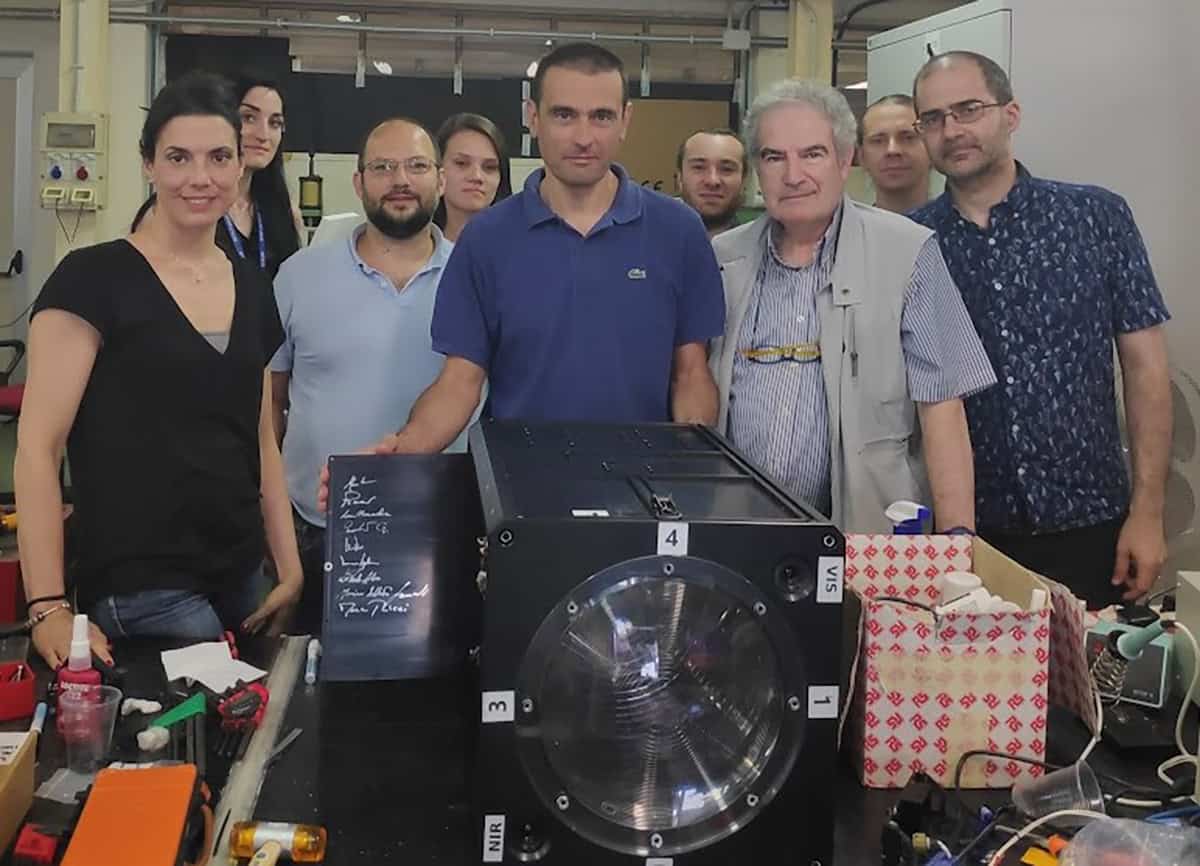
All of these experiments operate at night and require clear weather conditions, surveying regions of the sky with low-artificial-light backgrounds. In each case, the instruments in question monitor Earth’s atmosphere by measuring the fluorescence emissions and Cherenkov light produced by extensive air showers. The fluorescence originates from the relaxation of nitrogen molecules excited by their interaction with charged particles in the air showers, while ultrahigh-energy particles traveling faster than light in the air create a blue flash of Cherenkov light (like the sonic boom created by an aircraft exceeding the speed of sound).
Operationally, because those two light components exhibit different durations – of the order of microseconds for fluorescence light; a few nanoseconds for Cherenkov light – they require dedicated detectors and acquisition electronics: multi-anode photomultiplier tubes (MAPMTs) for fluorescence detection and silicon photomultipliers (SiPMs) for the Cherenkov detectors.
The win-win of technology partnership
So where do things stand with JEM-EUSO’s implementation of current- and next-generation detectors? Among the programme’s core technology partners in this regard is Hamamatsu Photonics, a Japanese optoelectronics manufacturer that operates across diverse industrial, scientific, and medical markets. It’s a long-standing collaboration, with Hamamatsu engineers co-developing and supplying MAPMT and SiPM solutions for various JEM-EUSO experiments.
“We have a close working relationship with Hamamatsu’s technical staff in Italy and, through them, a direct line to the product development team in Japan,” explains Marco Casolino, a research director at the National Institute of Nuclear Physics (INFN), Structure of Rome “Tor Vegata”, and the co-principal investigator on JEM-EUSO (as well as project leader for Mini-EUSO).
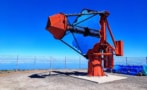
Silicon photomultipliers: gearing up for applications in gamma-ray astronomy
The use of MAPMTs is well established within JEM-EUSO for indirect detection of ultrahigh-energy cosmic rays via fluorescence (with the focal surface of JEM-EUSO fluorescence telescopes fabricated from MAPMTs). “Yet although MAPMTs are a volume product line for Hamamatsu,” Casolino adds, “the solutions we employ [for JEM-EUSO experiments] are tailored by its design engineers to our exacting specifications, with an almost artisanal level of craftmanship at times to ensure we get the best product possible for our applications.”
That same approach and attention to detail regarding JEM-EUSO’s evolving requirements also guide the R&D partnership around SiPM technology. Hamamatsu engineers are working to maximize the advantages of the SiPM platform, including significantly lower operating voltage (versus MAPMTs), lightweight and durable structure, and compatibility with magnetic fields. Another plus is that SiPMs are immune to excessive levels of incident light, although the cumulative advantages are offset to a degree by the strong influence of temperature on SiPM detection efficiency (and the consequent need for active compensation schemes).

Currently, JEM-EUSO scientists are focused on an exhaustive programme of test, measurement and calibration to optimize their large-scale SiPM detector designs – considering geometry, weight, packaging and robustness – for mass-critical applications in satellite-based instrumentation. “We are being very cautious with the next steps because SiPM technology has never been tested in space using very large detector arrays [e.g. 1024 x 1024 pixels],” explains Casolino. “Ultimately, it’s all about technical readiness level – ensuring that SiPM modules can handle the harsh environment in open space and the daily swings in temperature and radiation levels for the duration of a three- or four-year mission.”
Those priorities for continuous improvement are echoed by Massimo Aversa, senior product manager for MAPMT and SiPM product lines in Hamamatsu’s Rome division. “Our collaboration with JEM-EUSO is a win-win,” he concludes. “On the one hand, we are working to develop higher-resolution SiPM detector arrays with enhanced radiation-hardness – products that can be deployed for observations in space over extended timeframes. By extension, the lessons we learn here are transferable and will inform Hamamatsu’s SiPM development roadmap for diverse applications in high-energy physics.”
Further reading
Simon Bacholle et al. 2021 Mini-EUSO mission to study Earth UV emissions on board the ISS ApJS 253 36
Francesca Bisconti 2023 Use of silicon photomultipliers in the detectors of the JEM-EUSO program Instruments 7 55
SiPMs: versatile by design
The SiPM – also known as a Multi-Pixel Photon Counter (MPPC) – is a solid-state photomultiplier comprised of a high-density matrix of avalanche photodiodes operating in Geiger mode (such that a single electron–hole pair generated by absorption of a photon can trigger a strong “avalanche” effect). In this way, the technology provides the basis of an optical sensing platform that’s ideally suited to single-photon counting and other ultralow-light applications at wavelengths ranging from the vacuum-ultraviolet through the visible to the near-infrared.
Hamamatsu, for its part, currently supplies commercial SiPM solutions to a range of established and emerging applications spanning academic research (e.g. quantum computing and quantum communication experiments); nuclear medicine (e.g. positron emission tomography); hygiene monitoring in food production facilities; as well as light detection and ranging (LiDAR) systems for autonomous vehicles. Other customers include instrumentation OEMs specializing in areas such as fluorescence microscopy and scanning laser ophthalmoscopy.
Near term, Hamamatsu is also focused on emerging applications in astroparticle physics and gamma-ray astronomy, while further down the line there’s the promise of at-scale SiPM deployment within particle accelerator facilities like CERN, KEK and Fermilab.
Taken together, what underpins these diverse use-cases is the SiPM’s unique specification sheet, combining high photon detection efficiency with ruggedness, resistance to excess light and immunity to magnetic fields.