
Digital flat-panel detectors are central to today’s clinical X-ray imaging systems. But flat detectors are ill-matched to the complex 3D shape of a human body. A better option could be to use curved detectors, which could minimize distortion around image edges and reduce vignetting compared with their planar counterparts. Efforts to produce flexible detectors, however, have so far been unsuccessful, due to the stiff and brittle nature of the underlying inorganic (mainly silicon-based) semiconductor technology.
A promising alternative for creating curved X-ray detectors is to use hybrid “inorganic-in-organic” semiconductors. One candidate architecture comprises X-ray attenuating bismuth oxide nanoparticles integrated into an organic bulk heterojunction (BHJ) consisting of the p-type polymer P3HT and the n-type PC70BM.
For such a material to function effectively as a curved detector, it must combine high bendability with optimized detection performance. To achieve this, a team headed up at the University of Surrey has examined the influence of P3HT molecular weight on the mechanical and electrical properties of these nanoparticle-incorporated BHJ (NP-BHJ) X-ray detectors.
Reporting their findings in Advanced Science, the researchers found that by tailoring the molecular weight of the organic semiconductor to lengthen the polymer chains, they could create robust, high-sensitivity curved X-ray detectors for medical applications.
“Our curved detector concept has shown exceptional mechanical robustness and enables bending radii as small as 1.3 mm,” says lead author Prabodhi Nanayakkara in a press statement. “The use of organic or ‘inorganic-in-organic’ semiconductors is also far more cost effective than conventional inorganic semiconductors made from silicon or germanium, which require expensive crystal growth methods.”
Performance parameters
To investigate the impact of P3HT molecular weight on detector performance, Nanayakkara and colleagues created rigid NP-BHJ detectors using four P3HT molecular weights – 25, 37, 46 and 55 kDa – as typically employed in P3HT-based optoelectronic devices. They fabricated the detectors on glass substrates and incorporated 55 µm-thick NP-BHJ films.
The team assessed a series of parameters, including dark current, photocurrent characteristics and charge transport characteristics. The detector dark current decreased with reducing polymer molecular weight. All devices, however, displayed low dark currents well within the industrial requirement of 10 pA/mm2 at an applied bias from −10 to −200 V. The device sensitivity was slightly higher for the lowest P3HT molecular weight, with a limit of detection (for a 70 kV X-ray source) of around 1.5 µGy/s – better than required for diagnostic imaging.
While performance parameters differed between the four devices, the researchers emphasize that all P3HT molecular weights evaluated provided excellent detector response characteristics, including ultralow dark current, high sensitivity and fast response time, highlighting the potential of NP-BHJ detectors for low-dose imaging and dosimetry.
Optimizing bendability
Next, the team assessed the mechanical properties of various detector constructions. X-ray detectors require a higher crystallinity to achieve satisfactory charge extraction. But with curved detectors, higher crystallinity can result in mechanical failure. To identify the most suitable P3HT molecular weight for a curved X-ray detector, the researchers performed grazing incidence wide angle X-ray scattering measurements on NP-BHJ films.
They found that crystallinity was lower for films fabricated from higher P3HT molecular weights, suggesting that such films have more amorphous regions that can bridge between the crystalline regions. These amorphous regions also increase intermolecular interactions and interchain entanglements, enabling greater deformation before mechanical failure.
The researchers also examined the impact of substrate thickness on the nanomechanical properties of various NP-BHJ films. They fabricated detectors on flexible polyimide substrates with thicknesses lower than (25 µm), equal to (50 µm) and higher than (75 µm) the 55 µm NP-BHJ layer. The mechanical behaviour was dependent on both P3HT molecular weight and substrate thickness. Lower molecular weight detectors were stiffer and extremely brittle, while those fabricated on thinner substrates curled excessively and delaminated easily from the substrates.
Based on the crystallinity and nano-mechanical analyses, the researchers predict that higher P3HT molecular weights and thicker flexible substrates are most suitable for fabricating curved X-ray detectors. With this in mind, they tested the responses of three detectors – 46 and 55 kDa P3HT on 75 µm substrates, and 55 kDa P3HT on a 50 µm substrate – to 40 kV X-ray irradiation under different bending radii.
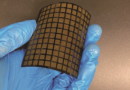
Flexible X-ray detector constructed without harmful heavy metals
All detectors displayed excellent resistance to bending, maintaining sensitivities of roughly 0.17 µC/Gy/cm2 and a dark current of less than 1 pA/mm2, even when curved to a radius as small as 1.3 mm. The team demonstrated the detectors’ mechanical robustness by performing cycles of dynamic bending down to a radius of 1.3 mm. After 100 cycles, the detectors exhibited less than 2.8% variation in sensitivity.
“The technology we’re demonstrating will help create a revolutionary new high sensitivity X-ray detector that is scalable, due to the design and materials adopted,” says senior author Ravi Silva. “This technology has huge potential in medical applications and other X-ray uses, so we’re working with a spinout company, SilverRay, and hope to turn this technology into the X-ray detector of choice for high-sensitivity, high-resolution, flexible large-area detectors.”