Recent observations may indicate a cut-off in the spectrum of ultrahigh-energy cosmic rays, which would imply that they originate from cosmologically distant sources.
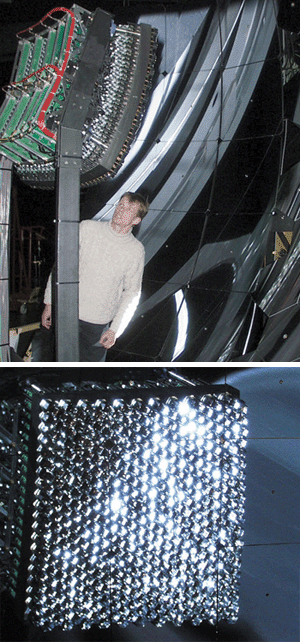
Physicists have known since 1912 that the Earth is constantly bombarded by a cosmic rain of high-energy particles – mostly protons and heavier nuclei – but until recently little progress had been made in understanding where these particles might come from. The reason we cannot do astronomy with cosmic rays is simple: they are charged particles that are deflected by magnetic fields, which means that we cannot determine where they originate from. Even the galactic magnetic field, which is only a few microgauss, can redirect cosmic rays that have energies up to 109 gigaelectron-volts (1018 eV) so that they appear to come uniformly from all directions.
Cosmic rays with ultrahigh energies do exist, although their number falls by a factor of about 1000 for every tenfold increase in energy, i.e. dN/dE is proportional to E-3, where N is the number of particles and E is their energy. Although these ultrahigh-energy cosmic rays are relatively rare, they are deflected less by magnetic fields. This means that we should be able to look back along their incoming directions and identify the astrophysical sites where these particles with such incredible energies are generated naturally. In contrast to these ultrahigh-energy cosmic rays, the Large Hadron Collider, which is currently being built at CERN, will accelerate particles to only about 104 GeV.
Interest in ultrahigh-energy cosmic rays grew following the serendipitous discovery in 1965 of the cosmic microwave background – fossil radiation left over from the hot Big Bang. Soon afterwards it was realized that if the energy of a cosmic-ray proton exceeded 5 x 109 GeV then, in its rest frame, a typical cosmic-background photon would be Lorentz-boosted to gamma-ray energies high enough to excite the proton. Cosmic-ray protons above this energy threshold – known as the Greisen-Zatsepin-Kuzmin (GZK) cut-off – would lose energy rapidly by emitting pions, leading to a sharp drop in their numbers. Such a cut-off would occur only if the sources of such ultrahigh-energy particles are cosmologically distant.
Conversely the observation of particles with energies above the GZK limit would imply that the sources are located in our cosmic backyard. Detailed calculations confirm that most of the cosmic rays observed above the GZK energy must originate within the local supercluster of galaxies, which is typically about 100 million light-years across.
Detecting cosmic showers
Remarkably the first particle with an energy of 1011 GeV was discovered by John Linsley of the University of New Mexico at the pioneering Volcano Ranch experiment several years before the discovery of the cosmic microwave background. At such huge energies the interaction of the primary cosmic ray with air molecules in the atmosphere triggers an “air shower”. This disc-like ionization front travels close to the speed of light and spreads out to cover an area of tens of square kilometres by the time it hits the ground.
The total number of secondary particles in an air shower can exceed the number of stars in our galaxy. Fortunately we only need to measure the particles within 600 m of the core of the shower to estimate the energy of the primary cosmic ray with an accuracy of about 30%. Such estimates are obtained from detailed comparisons with computer simulations of the shower. At these extreme energies, however, the probability of proton-air collisions must be estimated by extrapolating low-energy measurements made in the laboratory with the help of theoretical models.
Unfortunately although a great deal has been learned about high-energy particle collisions from experiments at accelerators, the interests of particle phenomenologists and cosmic-ray physicists are literally orthogonal. Phenomenologists are mainly interested in “hard” collisions that produce particles with large transverse momentum – these collisions are well understood using the theory of perturbative quantum chromodynamics. Meanwhile cosmic-ray physicists are concerned with the much more frequent “soft” events, which are usually non-perturbative and thus much harder to model accurately.
A breakthrough came with the Fly’s Eye experiment, which tracked cosmic rays through the atmosphere by observing the faint ultraviolet fluorescence that is produced when particles in the air shower excite nitrogen molecules. In 1993 the experiment, which was operated by the University of Utah, measured an event that had an energy of 3 x 1011 GeV – equivalent to the kinetic energy of a Pete Sampras serve on a single proton!
Several controversial events had previously been observed, in particular by the Haverah Park array in Yorkshire and by the Yakutsk array in Siberia, but since the Fly’s Eye measurement was direct, and based on using the Earth’s atmosphere as a gigantic calorimeter, it laid to rest doubts about the existence of ultrahigh-energy cosmic rays. Confounding some expectations, the Fly’s Eye experiment demonstrated that none of the events observed beyond the GZK energy could be traced back to any plausible nearby astrophysical source – the uniform distribution of these ultrahigh-energy cosmic rays therefore suggested instead that the sources are cosmologically distant.
The mystery continued to deepen with further observations using the Akeno Giant Air Shower Array (AGASA) in Japan, which recorded many events with energies well above the GZK energy (see figure 1). The AGASA collaboration measured a clear excess of ultrahigh-energy cosmic rays compared with the spectrum expected for distant sources.
Exotic and ordinary explanations
When reviewing the situation back in 1998, Michael Hillas of Leeds University emphasized that the simplest location for sources that are both nearby and isotropically distributed is the halo of our galaxy. The same proposal had been made independently by Venya Berezinsky and Michael Kachelreiss of the Gran Sasso Laboratory in Italy along with Alex Vilenkin of Tufts University in the US, and by Mike Birkel and the current author at Oxford University. We had suggested that ultrahigh-energy cosmic rays are generated by the slow decays of supermassive dark-matter particles that are predicted by unified theories, and that they could have been created in the early universe. Although such dark-matter particles are well outside the Standard Model of particle physics, the energy spectrum of their decay products is essentially determined by the theory of quantum chromodynamics together with data describing the decay of heavy particles, such as the Z boson.
Our calculation gave a reasonable fit to the AGASA data beyond the GZK energy and predicted the mass of the dark-matter particles to be in excess of 1012 GeV, much greater than all the known particles. Interestingly this is the same energy scale as the “hidden sector” in supersymmetric extensions of the Standard Model, and such particles, named cryptons by John Ellis of CERN, had in fact been predicted to exist with the required long lifetimes. Moreover, in this model cosmic rays cannot bombard the Earth by exactly the same amount in all directions because we are not at the centre of the galaxy – a characteristic that can be tested with more data.
Now John Bahcall of the Institute of Advanced Studies in Princeton and Eli Waxman of the Weizmann Institute in Israel have challenged whether such exotic physics is in fact necessary (arXiv.org/abs/hep-ph/0206217). They point out that the HiRes experiment, the successor to the Fly’s Eye array, has found far fewer events (so far unpublished) above the GZK energy even though it has had a similar exposure to cosmic rays as AGASA. By considering just the data from the air-fluorescence detectors (i.e. Fly’s Eye and HiRes) and the Yakutsk array, they argue that there is indeed a GZK cut-off, and that this implies that the sources are at cosmological distances (see figure 2).
Bahcall and Waxman’s preferred sources are gamma-ray bursts, which are believed to be the most violent explosions in the universe that release an energy equivalent to the rest mass of the Sun within a few seconds or minutes. They point out that the “relativistic fireball” model of gamma-ray bursts incorporates strong, collisionless shocks that could accelerate cosmic rays to 1011 GeV . Nevertheless the energy requirements are formidable. The gamma-ray bursts would have to put three times more energy into accelerating 1010-1011 GeV protons than they are observed to generate as gamma rays. In this model the gamma rays are emitted as synchrotron radiation by electrons that are accelerated to much lower energies.
However, cosmic-ray experimentalists are sceptical about this claim. Alan Watson at Leeds, who is spokesperson for the Pierre Auger Observatory in Argentina, notes that the Yakutsk array has been plagued by a problem that has prevented it from recording very energetic events. Emphasizing the Yakutsk data while ignoring the AGASA data, as Bahcall and Waxman have done, is therefore not advisable.
Moreover, the Fly’s Eye and HiRes experiments establish the shower energy from the observed fluorescence of nitrogen molecules. This determination is based on a measurement of the efficiency of the process that is nearly 40 years old and may need to be revised.
Complex corrections must also be made to take into account the state of the atmosphere, and this is more reliably done with “stereo” observations of the air showers with two well-separated detectors than “mono” observations, which account for the bulk of the reported exposure. In fact, in 1999 the HiRes collaboration reported a number of events beyond the GZK cut-off that were consistent with the AGASA data, but the researchers subsequently withdrew the result after recalibrating the energy measurements. Thus the discrepancy is really between the two methods of studying air showers: measuring the shower’s pattern on the Earth’s surface, as with the Volcano Ranch, Haverah Park, Yakutsk and AGASA arrays; and measuring the fluorescence along the shower’s path through the atmosphere, as the Fly’s Eye and HiRes teams did. It is therefore essential for measurements to be made simultaneously using both techniques to assess the problem and to move the field forward.
This is precisely what will take place at the Pierre Auger Observatory, which saw “first light” last year. Within a few years the question posed in the title of this article should have a clear answer. Looking further ahead, the European Space Agency’s Extreme Universe Space Observatory, which is scheduled to fly on the International Space Station in 2008, will use the atmospheric-fluorescence technique to monitor a huge area and boost the statistics of ultrahigh-energy cosmic rays considerably.
We will soon know whether the solution to the mystery of ultrahigh-energy cosmic rays is novel astrophysics or physics far beyond the Standard Model.