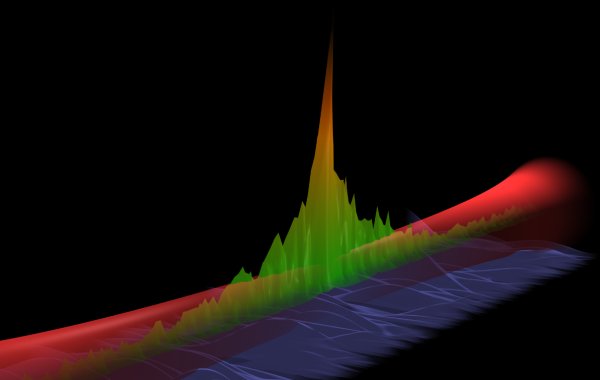
Two independent teams of physicists have used ultracold atomic gases to demonstrate how a little bit of disorder can paralyse a quantum system — an effect called “Anderson localization”. Their experiments mark the first time that this phenomenon has been seen in matter, despite being predicted to occur in crystalline solids 50 years ago.
Although the experiments were done in 1D optical lattices with non-interacting atoms, the teams claim that their methods could be expanded to investigate 2D and 3D systems or to study the effects of interactions on localization. This could allow such systems to be used as “quantum simulators” to gain insight into real materials that are difficult to probe experimentally or simulate numerically.
Much of what we know about the electronic properties of metals and semiconductors is based on the idea that electrons with certain momenta can travel freely through a crystalline lattice, while others cannot. This is embodied in Felix Bloch’s 1928 quantum theory of conduction, which describes the lattice as a periodic electric potential through which some electrons (behaving as “matter waves”) diffract with ease.
Disordered lattice
Some 30 years later, Philip Anderson worked out what would happen in such a system if the potential lost its periodicity. This could happen, for example, if the lattice remained periodic, but the potential has a different value at each lattice site. He found that electrons would be unable to move through such a “disordered” lattice, and instead become trapped by specific atoms. This prediction of “Anderson localization” won him the 1977 Nobel Prize in Physics.
Although Anderson localization has been seen in a number of systems — including those based on light and microwaves — it has never been observed directly with electrons. This is because lattice vibrations and interactions between electrons that occur in real material washes out the subtle effects of localization.
Now, two independent groups claim to have seen Anderson localization in matter waves for the first time. Both teams saw the phenomenon in Bose–Einstein condensates (BECs) made from ultracold atomic gases. In both systems it was individual atoms — rather than electrons — that became localized. Instead of being in a solid crystal, the atoms were in an optical lattice created by overlapping laser beams.
Speckled pattern
In the first experiment, Alain Aspect and colleagues at the University of Paris-Sud and CNRS’s Institut d’optique began by confining about 17,000 rubidium atoms in a magnetic trap to make a BEC (Nature 453 891). Turning the fields off makes the atoms quickly leave the centre of the trap. But if the BEC is placed in a lattice created by a laser “speckle” pattern — a random distribution of light and dark regions that is created when a laser beam is reflected from a rough surface — the atoms stay put when the magnetic fields are turned off. The team claims this effect is caused by Anderson localization.
Phllippe Bouyer at Paris-Sud told physicsworld.com that the team is now looking at how to repeat their experiment in 3D — something that will require a major redesign to overcome (among other things) the effect of gravity pulling down on the atoms.
Meanwhile in Italy, Massimo Inguscio and colleagues at the University of Florence began with a 1D optical lattice that is a standing wave created by an intense laser beam (Nature 453 895). If a second, weaker laser with a different wavelength is shone into the lattice, the two beams interfere to create a 1D “quasicrystal” with a certain amount of disorder. The degree of disorder in the quasicrystal can be adjusted by changing the intensity of the second laser.
The team then loaded the centre of their lattice with potassium atoms and watched what happened for different degrees of disorder. Sure enough, when the second laser was switched off, some of the atoms began to diffuse away from the centre of the lattice, finding it easy to move through the periodic potential. However, when the experiment was repeated with the second laser switched on, Anderson localization kept the atoms in the centre of the lattice.
Interacting atoms
The Florence team are planning to repeat their experiment with interacting atoms. Team member Giovanni Modugno told physicsworld.com that this will be done by adjusting the applied magnetic fields that the team currently use to eliminate interactions between atoms — a phenomenon known as “Feshbach resonances”.
Daniel Steck, a physicist at the University of Oregon, told physicsworld.com that “these experiments with cold atoms can certainly help in the study of disordered media”. He added, “in real materials, it’s very difficult to see coherent quantum effects directly, such as localization”.