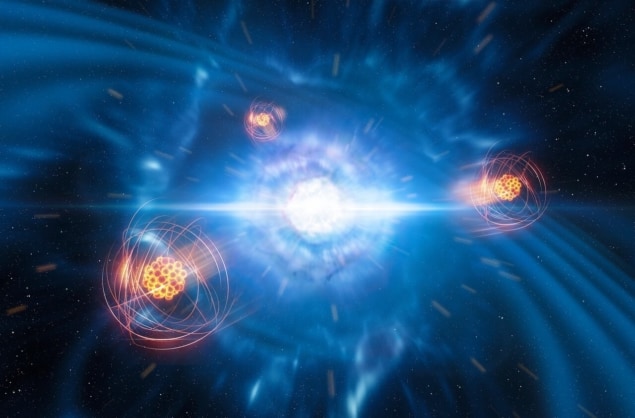
The mystery of where heavy elements such as gold and silver come from has deepened with research groups clashing over whether binary neutron-star mergers can account for the abundance of those elements in the universe.
On 17 August 2017 a burst of gravitational waves was detected by the LIGO and Virgo detectors. Astronomers quickly turned their telescopes towards the source of the waves and observed the afterglow of a kilonova – the collision of two neutron stars – in a galaxy 140 million light-years away.
The light of the kilonova was powered by the radioactive decay of large amounts of heavy elements formed by rapid neutron capture (the “r-process”). In particular, a team led by Darach Watson at the Niels Bohr Institute at the University of Copenhagen identified the spectral line of strontium in the kilonova’s light.
Enough collisions
It is now certain that neutron-star collisions produce r-process elements such as strontium, europium, silver and gold. However, debate continues as to whether there are enough such collisions to produce the abundance of those elements that we observe in the universe.
In a paper submitted to Monthly Notices of the Royal Astronomical Society, astronomers led by Irina Dvorkin of the Institut d’Astrophysique de Paris studied how the interstellar medium becomes enriched in r-process elements and concluded that binary neutron-star mergers are the main source of these elements in environments that have generally low levels of heavy elements. These regions include the Milky Way’s halo, dwarf galaxies and the early universe. Although their models did not focus on environments richer in heavy elements, such as the Milky Way’s disc, they propose that binary neutron-star mergers could also be the dominant source there too.
One of their reasons for this conclusion is the observed variations in the ratios of r-process element abundances compared to iron-group elements, which are formed in supernovae. If r-process elements were also formed in supernovae, we would expect them to have a constant ratios relative to the iron-group elements. Instead, there is a large scatter observed in the ratios of the two element groups, implying different origins.
Timescale too long
However, this conclusion is disputed by a paper in The Astrophysical Journal by Chiaki Kobayashi of the University of Hertfordshire and team. Kobayashi and colleagues created models describing the origin of every element in the universe as a function of time and environment. They came to the conclusion that the timescale of binary neutron stars forming from supernovae and then spiralling into a collision is too long to explain the observed abundance of r-process elements.
“The important difference between our paper and the Dvorkin et al. paper is the time delay of neutron-star merger events,” Kobayashi tells Physics World. Following their formation, binary neutron stars could take billions of years to get close enough to collide. However, some binary neutron stars may be able to merge much faster; for example, PSR J0737-3039 – the only double pulsar discovered so far – is a relatively young binary neutron-star system that will merge within a timescale of 85 million years.
Dvorkin adds, “In my opinion, the fraction of fast mergers needs to be further studied, as it’s clearly central to the question of the early production of heavy elements.”
If a significant number of binary neutron stars can undergo fast mergers, it will speed up the rate at which they enrich the interstellar medium – the gas and dust between stars – with r-process elements.
Watson, who made the key strontium discovery in the kilonova afterglow, acknowledges that observations are currently contradictory. “The amount of material produced in small dwarf galaxies [with low heavy-element abundances] is, I believe, largely inconsistent with neutron star production,” he says. “However, I also believe that it may be possible for neutron stars to merge on fast timescales.”
Sub-micron diamonds
Watson points out another line of evidence not considered in the Dvorkin or Kobayashi papers: nanodiamonds. These are tiny, sub-micron diamonds that can form in a variety of environments in space, from star-forming regions to asteroid collisions, but some nanodiamonds also contain r-process elements. Since neutron-star collisions do not produce nanodiamonds, the only other possible source of r-process enriched nanodiamonds is supernovae, says Watson.
Although the scatter in the ratios of r-process elements to iron-group elements observed by Dvorkin’s team seems to rule out ordinary supernovae, Kobayashi proposes a new type of exploding star called a magneto-rotational supernova. However, there is no direct evidence for the existence of such supernovae and the debate regarding the origin of r-process elements will continue to rumble on.