Physicists at the Massachusetts Institute of Technology (MIT) in the US have found a new way to switch antiferromagnetism on and off. Their technique, which involves doping antiferromagnetic materials with extra electrons, could allow for faster, higher-density and more stable memory storage.
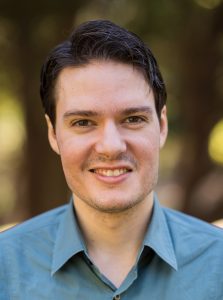
Modern hard disk drives encode data by using magnetic field pulses to flip the spins of electrons (representing binary zeros and ones) in ferromagnetic materials within computer chips. While researchers have found ways to switch these binary bits at ever-smaller scales, the magnetic pulses require a relatively large electrical current, so the data-writing process dissipates a lot of energy. It is also relatively slow, with a complete spin flip taking tens of nanoseconds.
Promising candidates
Antiferromagnetic materials are promising candidates for future high-density memory applications because the spins in antiferromagnets flip much faster, at frequencies in the terahertz range. These rapid spin flips are possible because of strong interactions between electron spins, which come about because the spins in antiferromagnets tend to align so that they are antiparallel to each other (unlike conventional ferromagnets, which have parallel electron spins).
Antiferromagnets also lack magnetization on the scale of a single bit (>10 nm), making them robust to external magnetic perturbations. This means that data encoded in a memory device based on antiferromagnetic bits could not be erased magnetically. A further advantage is that antiferromagnets could be made into smaller transistors (and thus be packed more densely onto chips) than is possible with conventional transistor materials like silicon.
Creating oxygen vacancies
In the new work, MIT researchers led by Riccardo Comin set out to determine whether they could manipulate the antiferromagnetic properties of 100-nm-thick films of samarium and neodymium nickel oxide (SmNiO3 and NdNiO3) by doping them with extra electrons. While doping usually involves introducing impurities in a material to alter its electronic properties, in this case Comin and colleagues achieved what’s known as carrier doping by stripping the SmNiO3 and NdNiO3 of oxygen atoms. This process leaves two electrons behind for every oxygen removed, and the left-behind electrons redistribute themselves among the remaining oxygen atoms and the nickel.
During these experiments, the researchers monitored the films using advanced magnetic X-ray crystallography techniques to determine whether the material’s magnetic structure remained intact – in other words, whether the atomic spins retained their orderly anti-alignment and therefore their antiferromagnetism. Any reduction in the previously ordered magnetic structure, they explain, would be evidence that the doping was enough to switch off the material’s antiferromagnetism.
Sharp magnetic transition
The researchers say the antiferromagnetic order in rare earth nickelates like the ones they studied is remarkably robust to carrier doping – up to a point. Then, at a critical doping threshold of 0.21 electrons per nickel atom, the order suddenly collapses. This magnetic transition is sharp, similar to switching a transistor’s state from zero to one, and can be reversed by adding oxygen back into the material.

Light-induced lattice vibrations could speed up data recording
Comin says that, like conventional ferromagnetic bits, antiferromagnetic bits might be switched using voltage gates (a process in which a small voltage is applied to a bit to alter its electrical conductivity). He tells Physics World that this would allow rare earth nickelate materials to be employed in field-effect devices that could directly read/write operations on antiferromagnetic bits.
The researchers, who report their work in Physical Review Letters, say they now hope to gain better control over the magnetic switching process, optimize it for device applications and explore more exotic physical properties in the rare earth nickelate family for functional applications. They also plan to study other materials with potentially higher magnetic transition temperatures as potential platforms for a new magnetic storage technology.