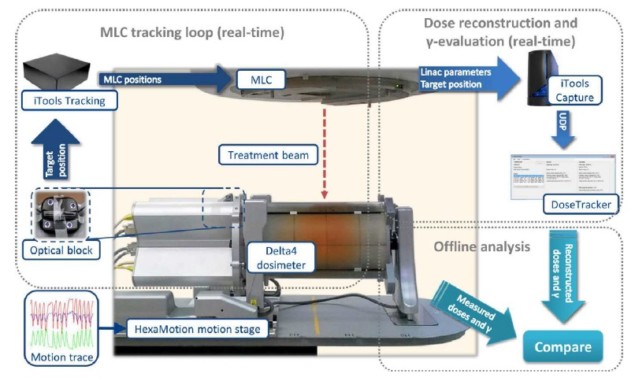
Danish researchers have developed and validated a four-dimensional (4D) dose reconstruction and evaluation system that tracks radiation dose delivered to abdominal or thoracic tumours affected by motion. The system represents the first real-time, delivery-specific quality assurance (QA) performed at a linear accelerator during radiation delivery to moving targets (Med. Phys. 10.1002/mp.13037).
Performing QA of treatment plans adapted for anticipated tumour motion is a time-consuming process, and does not guarantee accuracy since the tumour motion may differ during treatment. A better approach would be to perform the QA during treatment using the actual tumour motion. As such, the team, from Aarhus University Hospital, developed the system specifically to monitor radiation dose delivery errors caused by tumour motion during radiation delivery.
The system, named DoseTracker, is designed to work with both tracking and non-tracking systems on conventional linear accelerators. DoseTracker is based on a fast motion-including (FMI) dose algorithm, which is a hybrid between a series of point calculations and a pencil beam convolution algorithm.
For dose evaluations, principal investigator Thomas Ravkilde and colleagues used a spatially focused analysis, the γ-test of the cumulative dose, due to its usefulness at all times during beam delivery. They note that while dose-volume histograms (DVHs) could be a more clinically relevant measure, they might not be meaningful for a partially delivered fraction, and could obscure spatial distribution of dose peaks and valleys within a structure.
Summarizing how the system works, the authors explain that DoseTracker receives linac parameters and target positions as unified data protocol (UDP) messages, and reconstructs the transient dose and cumulative dose to a phantom, with the measured motion (the actual dose) and without motion (the planned dose). An independent loop calculates the motion-induced γ error of the latest available cumulative dose distributions by comparing reconstructed doses with and without motion.
Positions of calculation points can be shifted independently between each transient dose reconstruction, to mimic translational, rotational or deformational motion. This capability is needed to ensure the accuracy of motion-including dose reconstruction.
System validation
The authors validated DoseTracker using the original five-field coplanar volumetric-modulated arc therapy (VMAT) plans of five patients with liver cancer, delivered with and without multi-leaf collimator (MLC) tracking to a motion stage carrying a Delta4 dosimeter. The liver motion during the treatments had previously been recorded by kilovoltage intrafraction monitoring (KIM). The motion stage reproduced the KIM-measured tumour motions in 3D, while optical monitoring guided the MLC tracking and provided the position signal to DoseTracker.
The researchers performed 90,560 actual and 90,560 planned dose reconstructions online and in real time, and 5237 γ evaluations, with median computation times of 30 ms and 1.2 s, respectively. The mean difference between reconstructed and measured doses was –1.2% for transient doses and –1.5% for cumulative doses. DoseTracker’s ability to predict γ–fail rates above a given threshold had a mean sensitivity of 96.8% and a specificity of 99.2%.
“DoseTracker accurately predicted the instantaneous validity of the ongoing radiation treatment throughout beam delivery with high frequency and high specificity for all clinically relevant γ–fail rate action thresholds,” the authors write. “Reconstructed doses and dose evaluations were all benchmarked and validated against simultaneous phantom measurements.”
Ravkilde and colleagues believe that their system will be appropriate for treatments with real-time motion adaptation by gating or tumour tracking and for treatments without motion adaptation. They suggest that DoseTracker will resolve an inherent problem associated with pre-treatment plan-specific QA for MLC tracking, which cannot determine MLC leaf positions of future fractions. DoseTracker would provide a seamless form of in-treatment QA at every fraction.
When used in treatments without motion adaptation, DoseTracker would alert for treatment interruption in cases of large motion-induced errors, or could provide dose-based gating instead of conventional geometrically-based gating.
“We have been preparing for clinical use of the system,” Ravkilde tells Physics World. “During validation and testing, we can use an infrared block meant for use on the patient surface, but this does not provide the internal motion of the tumour in a patient. So we have worked on integration of combined optical and sparse monoscopic imaging with kilovoltage X-rays with DoseTracker.”
The team’s aim is to provide real-time online motion-including dose evaluation during radiotherapy, on a conventional linear accelerator and using only widely available standard equipment. “We also aim to use DoseTracker for real-time QA in clinical trials of tumour tracking where all of the necessary information, including tumour motion, is readily available by definition,” says Ravkilde. The team hopes to reach these goals within a year.
The researchers have also been working to refine the algorithm for real-time calculations on patient anatomy. “Our preliminary work shows good results on patient anatomy, even with very few additions to the original algorithm. The most challenging problem to solve for real-time use on patient anatomy is adequate inclusion of tissue heterogeneity,” Ravkilde says.