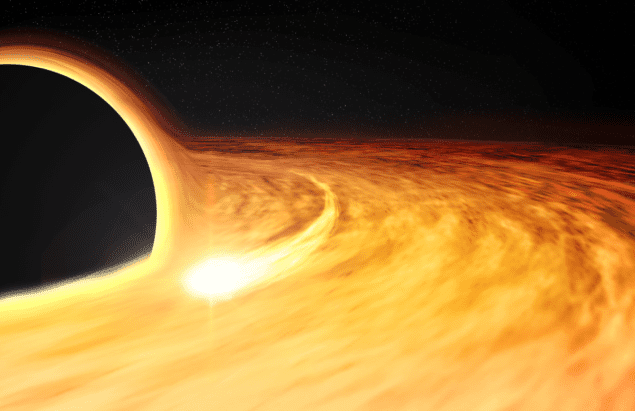
A surprising X-ray signal coming from a supermassive black hole at the centre of a distant galaxy could provide crucial information for determining how these behemoth objects are formed.
The two most fundamental properties of a black hole are its mass and its spin. If you know these, “then you know everything there is to know about a black hole,” says Dheeraj Pasham of the Kavli Institute for Astrophysics and Space Research at the Massachusetts Institute of Technology, who led the observation.
Measuring the mass of a supermassive black hole at the heart of a galaxy is relatively straightforward. Astronomers just determine the velocity of stars in orbits around the object, and the more massive the black hole, the faster the stars are moving. Measuring how a black hole is spinning, however, is more complex because the spin only affects space-time very close to the black hole’s event horizon.
X-ray flashes
On 22 November 2014, the All-Sky Automated Survey for Supernovae (ASASSN) detected a massive flash of X-rays from a galaxy 300 million light-years away. Called ASASSN-14li, the event proved not to be a supernova, but the death cry of a star pulled apart by the tidal gravitational forces of a supermassive black hole with a mass about a million times greater than the mass of the Sun.
A trio of X-ray satellites – the Chandra X-ray Observatory, XMM-Newton and Swift – quickly followed up, and while pouring through the data collected, Pasham and his colleagues in the United States and Europe found an additional, mysterious but periodic, X-ray signal that persisted for the 450 days of observations made by the three satellites.
The signal brightens and fades every 131 s and, at its peak, is 40% stronger than the average X-ray brightness of the black hole. The short period implies that it comes from a source that is orbiting very close to the black hole, in what is known as the innermost stable circular orbit (ISCO). This is the smallest orbit that an object can take around a black hole without falling through the event horizon. The faster the black hole is spinning, the closer the ISCO is to the event horizon. Based on the 131-second periodicity of the orbit, Pasham’s team calculate that the event horizon of the black hole is spinning at half of the speed of light.
Spaghetti and a white dwarf
Some of the debris from the dead star, which produced the original burst of X-rays when it was “spaghettified” by the black hole’s gravitational tidal forces, should still be circling around the black hole, but the debris alone would not produce the periodic X-ray signal. Instead, the best explanation, says Pasham, is that there is an unidentified object also in orbit around the black hole. This object must be denser than the star that was torn apart, otherwise it too would have been destroyed by gravitational forces – and this leaves few options.
Pasham’s theory is that the object in the ISCO is a white dwarf, which is the dense core remnant of another star. The stellar debris accumulates onto the white dwarf, creating a hotspot on its visible surface. As the white dwarf orbits the black hole, we see the X-ray signal from the hotspot periodically brighten and fade as it moves behind the black hole.
Pasham is cautious about this theory. “While this model can explain the observation, it is a very rare situation,” he tells Physics World. This is because the white dwarf should not linger in the ISCO for more than a few hundred years. Although the orbit is gravitationally stable, other forces are at work, These include the viscosity and turbulence of the stellar debris through which the white dwarf is moving, magnetic fields around the black hole, radiation pressure and even the emission of gravitational waves, all of which will eventually see the white dwarf’s orbit decay into the black hole. Therefore, the likelihood of one star being ripped apart at the same time as a white dwarf being present around the black hole seems slim.
Constant supply of stars
However, the centres of galaxies are hotbeds for star formation, so there is a constantly replenished reservoir of stars for supermassive black holes to gobble up. Furthermore, there are enough supermassive black holes in the universe that all-sky searches should be able to detect more similar events – called “tidal disruption flares” – which could lead to some exciting possibilities.
Understanding how supermassive black holes form and grow remains a longstanding puzzle and knowing how fast the objects are spinning will reveal their formation history. The fastest spinning black holes are rotating at close to the speed of light. For example, the black hole at the centre of the galaxy NGC 1365 has been measured to rotate at more than 80% of the speed of light. Black holes with such rapid rotations are thought to form mostly from the accretion of large amounts of gas, whereas black holes formed mostly from the mergers of smaller black holes, where angular momentum is being added to the system from random directions, end up spinning more slowly. The high spin rate of the black hole in the ASASSN-14li event indicates that it may have formed mostly from gas accretion.
By measuring the spins of many black holes from future observed tidal disruption flares, “we can build spin distributions of supermassive black holes at various redshifts, which would directly constrain models for the growth of supermassive black holes,” says Pasham.
The research is described in Science.