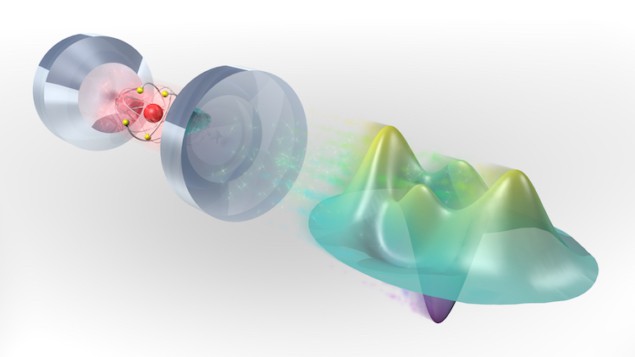
Home »
Quantum » Quantum optics » Light–matter entanglement creates Schrödinger-cat states
Light–matter entanglement creates Schrödinger-cat states
17 Jan 2019 Isabelle Dumé

Isabelle Dumé
is a contributing editor to Physics World