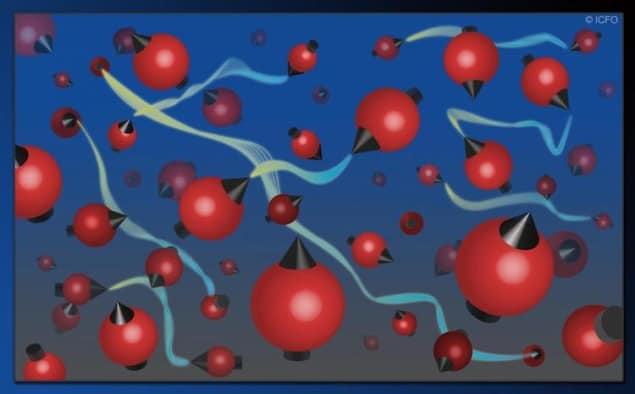
Entanglement – a purely quantum-mechanical effect that allows two or more particles to have a much closer relationship than classical physics permits – can survive high temperatures and chaotic environments. This unexpected finding from researchers at the ICFO in Barcelona, Spain could mean that entanglement-based quantum technologies, which were previously thought to function only in cold, low-noise conditions, may work in “hot and messy” environments too.
Quantum entanglement is the process by which particles such as photons become inextricably linked, such that if one is polarized in a vertical direction, then the other will always be polarized in a horizontal direction. Hence, by measuring the polarization of one photon in the pair, we immediately ascertain the polarization of the other, no matter how far apart they are. Once thought to be a quirky – or even nonsensical – aspect of the quantum world, this “spooky action at a distance”, as Albert Einstein called it, is now being exploited in quantum cryptography and quantum communications systems as well as sensors used to detect gravitational waves.
Entangled states are usually thought of as being extremely fragile. Even the tiniest disturbance (or noise) in their environment can cause entangled particles to “decohere” through random interactions, making the entanglement disappear. Current quantum technologies therefore typically operate at ultracold temperatures, and their designers go to great lengths to keep their quantum systems isolated.
The opposite strategy
The ICFO researchers, led by Morgan Mitchell, have now shown that the opposite strategy – actively promoting random interactions – can help generate and preserve entanglement too. In their experiment, they heated a collection of rubidium-87 (87Rb) atoms to 450 K, creating a vapour of hot alkali atoms. They found that individual atoms in this vapour were not isolated but collided with each other every 20 microseconds. Each collision set their electrons spinning in random directions, producing a magnetization.
Mitchell and colleagues used a laser to monitor this magnetization via a series of measurements that enabled them to detect entanglement between the atoms and study the effect of the atomic collisions. The measurement technique is known as optical quantum non-demolition (QND) because it can measure the electron spins without disturbing them. “If a regular measurement is like a biopsy, in which material is taken and analysed, then a QND measurement can be thought of as like MRI, in which we obtain information without damaging the system,” Mitchell explains.
Singlet-type entangled states
While many different types of collisions between atoms are possible, the most frequent are collisions in which the atoms exchange electron spin. The researchers observed that a huge number of rubidium atoms – at least 1.52 × 1013 out of 5.32 × 1013 participating atoms – were entangled via these spin-exchange collisions and entered singlet-type entangled states. “These are a curious state of two spins: each spin seems to be completely random, pointing in every direction simultaneously,” explains Mitchell. “Nonetheless, the spins always point in exactly opposite directions. It is thus a state that is completely coordinated while also being totally random.”
In the QND measurement, the contribution from a singlet is zero, he adds. “Since they point in opposite directions, what is contributed by one spin is exactly cancelled by the other spin. So, when we see the optical QND signal becoming very ‘quiet’, we know that many singlets have formed.”
And that was not all. As the ICFO team describe in their paper, which is published in Nature Communications, they also observed that the entanglement was non-local, meaning that it involves atoms that are not close to each other. Between any two entangled atoms there are thousands of other atoms, many of which are entangled with still other atoms, in a giant, hot and messy entangled state, they say.
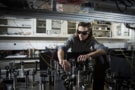
Energy-time entanglement detected in photons
Sensing technologies could benefit
When the measurement is stopped, the entanglement persists for about a millisecond, explains study first author Jia Kong. This means that a new batch of 15 trillion atoms is being entangled 1000 times per second. 1 ms is long enough for each atom to undergo about 50 random collisions, clearly showing that the entanglement is not destroyed by these random events. “This is maybe the most surprising result of our work,” she states.
The findings will be important for sensing technologies based on hot, dense clouds of atoms. One such technology, known as vapour-phase spin-exchange-relaxation-free (SERF) media, operates at 450 K and is used in applications as diverse as magnetometers that can detect magnetic signals from the brain and instruments that look for signs of dark matter and physics beyond the Standard Model. “Whether or not entanglement could survive at these hot temperatures was an open question until now, but our experiments show that it indeed can,” Kong tells Physics World.