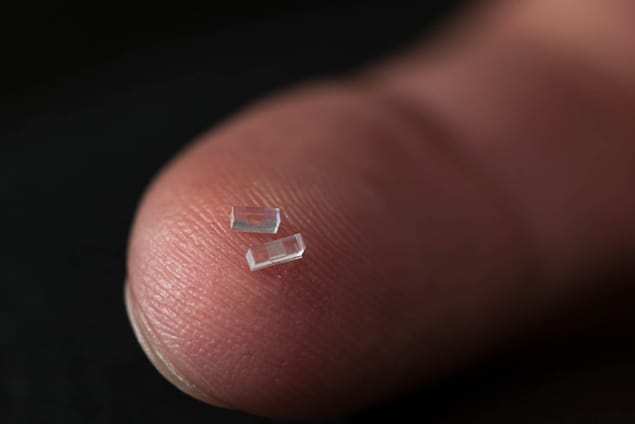
Two independent teams of physicists have used small pieces of glass etched with tiny gratings to accelerate electrons through enormous electric-field gradients. One team boosted the kinetic energy of the electrons at about the same rate as a conventional particle accelerator, while the other achieved 10 times that rate. The technology could one day be used to build table-top accelerators that are much smaller than conventional devices, bringing the benefits of particle-beam therapy to a wider range of cancer patients.
Laser-driven particle acceleration has been the subject of intense research over the past two decades, having been used to accelerate electrons, protons and other charged particles. Although several different techniques can be used, they all involve firing an intense pulse of laser light at a target. The intense electric field of the pulse separates electrons from the positively charged nuclei, creating a very strong electric field that can then be used to accelerate charged particles.
This latest breakthrough was made independently by two groups: one in the US and the other in Germany. The US team was led by Robert Byer of Stanford University and included physicists at the SLAC National Accelerator Laboratory, the University of California, Los Angeles and the Tech-X Corporation. In their set-up, a beam of electrons is first accelerated to a kinetic energy of about 60 MeV moving at near to the speed of light using the Next Linear Collider Test Accelerator Facility at SLAC. The other team was led by John Breuer and Peter Hommelhoff of the Max Planck Institute of Quantum Optics in Garching, whose device works for much less energetic 28 keV electrons travelling at about one-third the speed of light.
Pillars and trenches
In the US experiment, the laser acceleration is carried out by first firing the electrons into a 500-μm-long device made from silica glass. The electrons travel along a narrow channel, the two opposing walls of which are covered by gratings of pillars and trenches (see figure above). As the electrons zoom down this channel, a pulse of intense 800 nm infrared light is fired at the gratings – twice the wavelength of the gratings themselves. The pulse interacts with the gratings such that the phase of its electric field is rotated by 180° as the light passes a grating pillar. The strength of the electric field is also enhanced in a similar periodic manner.
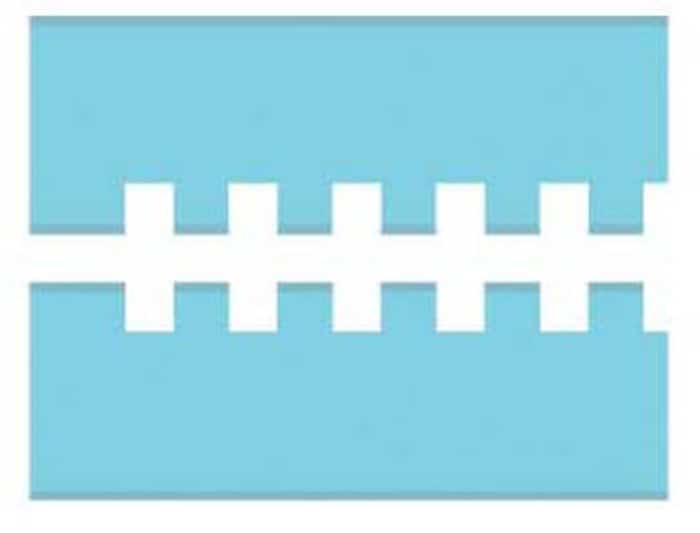
Some electrons enter the channel at just the right moment to experience a strong electric field that accelerates them in the forward direction. And because they are travelling at very nearly the speed of light, these electrons are synchronized with the pulse as it travels through the device – and therefore they enjoy maximum acceleration throughout their journey. The US team calculates that the electrons encounter an acceleration gradient of about 300 MV/m in the device – which is more than 10 times higher than that achieved in today’s conventional accelerators.
Non-relativistic challenges
The German device, in contrast, works for much slower electrons that travel a shorter distance in one oscillation cycle of the light pulse. This means that the grating spacing in the chip should be about 250 nm. As this was too small for the team to achieve, Breuer and Hommelhoff settled on a 750 nm spacing, which meant that the electrons got an accelerating kick once every three cycles. Furthermore, because the initial speed of the electrons is much lower than the speed of light, their speed will increase significantly when accelerated. So to be effective, the grating spacing must increase as the electron travels along the device.
Despite these problems, Breuer and Hommelhoff were able to create an acceleration gradient of about 25 MV/m, which is on a par with conventional accelerators. While these accelerators-on-a-chip could lead to compact sources of high-energy electrons for scientific, commercial and medical use, there are still challenges. In particular, the Stanford device works extremely well but requires a source of relativistic electrons – which is large and expensive. While Breuer and Hommelhoff have shown that it is possible to accelerate non-relativistic electrons, much more work would be needed to create a practical system that uses lasers to make a truly compact high-energy source.
However, that has not deterred Byer. “Our ultimate goal for this structure is 1 GV/m, and we are already one-third of the way there in our first experiment,” he says.
The work by Byer and colleagues is reported in Nature and Breuer and Hommelhoff describe their research in Physical Review Letters.