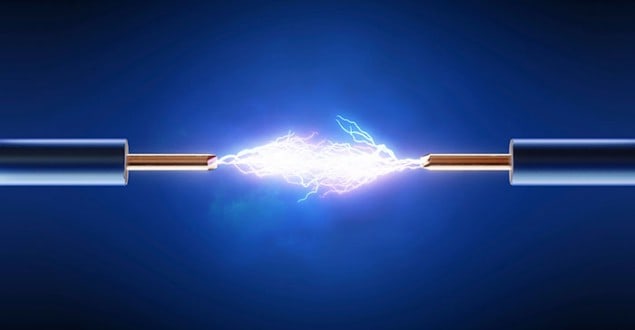
A room-temperature “supercurrent” has been identified in a Bose–Einstein condensate of quasiparticles called magnons. That’s the finding of an international team of researchers, which says the work opens the door to using magnons in information processing. Other researchers, however, believe the claim is premature, arguing that less-novel explanations have not been ruled out.
The term “supercurrent” describes the resistance-free current of charged particles in superconductors. It also describes the viscosity-free current of particles in superfluid helium. The common denominator of these systems is that they can be described as Bose–Einstein condensates (BECs) – collections of bosons, such as Cooper pairs or Helium-4, that can be described by a single wavefunction.
Frictionless flow
In such systems, the frictionless particles flow continuously. What causes the particles to move is not some external force – supercurrents endure without needing any input force to drive them. Instead, the flow is caused by a gradient in the phase of the BEC wavefunction. Previously, all examples of supercurrents have been at cryogenic temperatures. That’s because heat tends to excite particles into higher quantum states, which would destroy a BEC. The collection of particles would no longer be described by a single wavefunction, which would make macroscopic quantum phenomena such as supercurrents impossible.
In 2006, however, a team led by Sergej Demokritov at the University of Münster and Burkard Hillebrands of the University of Kaiserslautern, both in Germany, made a breakthrough that would pave the way for the new result reported here. The team showed that it is possible to create a BEC of magnons – quasiparticles which are quanta of electron-spin waves – at room temperature. To do this, the researchers applied a process called parametric pumping to a crystal film of yttrium iron garnet – a technique that injected magnons into the crystal’s ground state. They found that, when the magnon density became sufficiently high, the magnons formed a BEC.
Room-temperature first
In the new research, Hillebrands and colleagues produced such a magnon BEC, then heated its centre using a laser pulse. By changing the duration of the pulse, the researchers could alter the temperature difference they created between the laser-lit spot and the rest of the material. The purpose of the heating was to affect the magnetic properties of the material, and so affect the phase of the wavefunction governing the BEC.
The researchers observed the movement of magnons away from the heated region, and found that the magnitude of this flow increased with the temperature difference. Theoreticians in Ukraine and Israel constructed mathematical models of this flow, and believe that it can only be explained by the existence of a magnon supercurrent. “When we first saw it, we didn’t understand it,” says Hillebrands. “Fortunately, we have a very good team including top-level theoreticians.”
The researchers suggest that, in addition to fundamental scientific interest, the work could potentially lead to real-world applications. They say that they have opened the door to using room-temperature magnons for information storage and processing. “If we can now move this into a device where a macroscopic quantum state can be used, then for the future this looks very promising,” says Hillebrands. “This is at the very beginning – I cannot promise a device yet, but it is certainly very worthwhile to look into this new field.”
Insufficient evidence?
Demokritov, however – who was involved in the 2006 work but not this latest research – is sceptical that the researchers have genuinely observed a supercurrent. He believes the researchers have insufficient evidence that the flow is not simply due to the parametric pumping adding energy into the system. He offers the example of a magnetic stirrer, in which a rotating magnetic field turns a bar to stir a fluid. “If you looked at this, and you saw the fluid was rotating persistently, you might say that you had superfluidity at room temperature,” he says. “Of course, this would definitely not be the case because the energy would be brought to the system by the rotating magnetic field underneath the glass. I am afraid, unfortunately, that that is the case here.”
Demokritov also claims that his own team had better experimental evidence for a supercurrent – reported in 2012 in Scientific Reports – than that presented by Hillebrands and colleagues. “We were not so brave as to call it a supercurrent because the issue of dissipation is still open,” he says.
Demokritov is not the only sceptic. In July, physicist Edouard Sonin, at the Hebrew University of Jerusalem, Israel, published a comment on the arXiv preprint server in response to Hillebrands and colleagues’ paper, a pre-print of which was also first posted to the arXiv. He wrote that their pre-print “has not provided persuasive evidence of spin supercurrent” and that the authors neither checked the criteria for existence of a spin supercurrent in yttrium-iron-garnet magnetic films, nor did they discuss the “more natural scenario” that spin transport in their experiment was purely due to dissipation.
Hillebrands maintains that standard magnon-dissipation processes cannot properly explain the phenomena that his group have observed, although he concedes that they “still need to prove” that the flow is completely free of dissipation.
The research is published in Nature Physics.